World Food Supply and Biodiversity
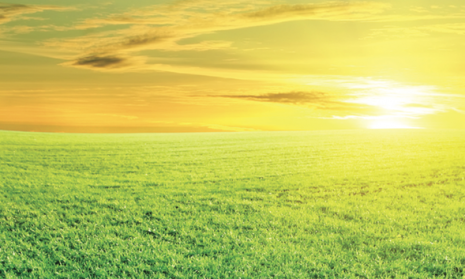
Summary
The current world population of around seven billion is projected to reach nine billion in forty years or less. The UN projects agricultural output will need to increase by 70% just to maintain current dietary standards – which includes more than one billion malnourished people. Current agricultural production is increasing at a rate insufficient to reach the goal set by the 2009 World Summit on Food Security to reduce by one half the number of malnourished people in the world by 2015. In spite of declining poverty rates, achieving this reduction in the number of malnourished people, even by 2040, will be very difficult as it is likely that the projected two billion additional people will be amongst the poorest of the poor. Food imports, worldwide, are expected to increase despite projected increased production. The required increase in world food production is possible on the area of land currently under production, whilst our fragile environment is protected.
Glossary
BT: a billion metric tonnes, i.e. 1012 kg;
cM: a unit of recombination where one map unit = 1 centimorgan (cM)
Introduction
It took until approximately 1932 for the human population to reach three billion people; it then took from 1932 to 1999 to reach six billion people. The latest United Nations-Food and Agriculture Organization (UN-FAO) projection is for the world to reach around nine billion people in 2040 (UN-FAO FAOSTAT, 2010), placing tremendous pressures on increasing world food production of all kinds. The UN projects that by 2050 agriculture will need to increase production by 70% (UN-FAO FAOSTAT, 2010) in order to maintain the same dietary standard we have today, but the world will still have more than one billion severely undernourished people and more than 100 million living near starvation.
Since 1990, the undernourished population has risen by around 9% on a global scale in spite of a 12% increase in world food production per capita (Barrett, 2010). Unfortunately, agriculture production is projected to increase by approximately 1.3% per year over the period including 2030 (Mann, 1999), therefore agricultural output will not come close to reaching the 2009 World Summit on Food Security goal of reducing by 50% the number of hungry and malnourished people in the world by 2015 (UN-FAO FAOSTAT, 2010). If true, the number of hungry and/or undernourished people in the world will not change, or most likely will increase.
The World Bank (2008) estimated that in 1990 approximately 1.13 billion people worldwide were living on less than $1 per day. In spite of all our technology and agricultural improvements, in 2010 roughly one billion people still face hunger on a daily basis. However, one must look very closely at the numbers involved. Many claim that there is no longer a major food problem, as currently evidenced by improvements in developing countries’ middle classes and a decrease in the percentage of poor people. This is true if we look at the percentages, but instead, we need to look at the numbers, since there are approximately one billion (14%) impoverished people today when the world population is approximately seven billion but when the world population reaches nine billion people it is projected that there will still be more than 1 billion poor people (11%) – doubtless an underestimate. Even with the projected increase in food production, food imports on a world scale will continue to increase; for example, wheat (Triticum aestivum L.) imports are projected to increase from 30 to 75 million tonnes (MT) by 2020 (Pingali and Rosegrant, 1998). It is clear that most of the two billion people who will be added to the world population in the next three decades will probably be among the poorest of the poor (Bruinsma, 2003), and hence the situation will become worse unless extraordinary improvements in food production are achieved in the near future.
Some 10 500 years ago, the human population consisted of about three to four million people. With the development of agriculture, first in the ‘Fertile Crescent’ of the eastern Mediterranean and then independently in other parts of the world, the human population began to grow rapidly. Domestication of the major food crops is the main reason for the emergence of human civilization as we know it today. In a period of just over 400 generations the majority of changes that we now regard as the core of human civilization took place. Considering that human beings have inhabited the Earth for approximately two million years, it is clear that we have been the dominant species for only a very small fraction of the history of our planet.
As agriculture intensified, the human population grew as it transformed from a world of hunter-gatherers to one in which towns, cities, and countries competed with one another for food supplies and arable land.
Plants, once domesticated, have been continually improved by selecting those most productive for sowing. The UN-FAO (UN-FAO FAOSTAT, 2010) has been documenting the improvements in agricultural food production since 1963 when about 2.63 billon metric tonnes (BT), including both plant (2.49 BT) and animal (0.14 BT), were produced. The latest UN-FAO data showed a phenomenal increase in world food production over the past 44 years to about 8.22 BT of total food production including both plant (7.07 BT) and animal (1.15 BT) in 2007 on basically the same amount of land (USDA-NASS, 2010). The dramatic increase in world food production, which supplies feed for animal production and food for human consumption, has mainly come from improved crop cultivars, technological advances, and management practices. Over the next 40 years around 80% of the required increase in world food production is projected to result from yield increases (67%) and higher cropping intensities (12%), with the remaining 21% coming from a minimal expansion of arable land under crop production (World Bank, 2008).
The main objectives in feeding the world’s population include, first, the task of increasing world food production and improving dietary standards of the chronically undernourished and the expanding population. Second, even if agriculture can accomplish the daunting task of increasing production, we will have to deal with the overwhelming task of equitably distributing food to all regions of the world to offset the current consequences of population growth and increasing world hunger. We will never see a lasting solution to the world food/hunger and poverty problem without a strong balance between food production and distribution – in other words – social justice. Third, agriculture needs to accomplish these objectives with a minimum impact on the world’s biodiversity and our fragile environment. Fourth, the increased food production needs to be accomplished without significantly expanding existing levels of land under cultivation.
Approximately 11% of the world’s landmass is used for crop production, with an estimated 22% more used for pasture, most of which is natural grassland and manifestly unsustainable for crop production. No more than 10% of additional arable land is potentially available even for limited crop production (Bruinsma, 2003). The massive increase in food production between the years 1963 to 2007 was accomplished utilising about the same amount of land currently under production (USDA-NASS, 2010). For example, world grain yields more than doubled from 1.3 MT per hectare in 1961-63 to 2.77 MT per hectare in 1997-99, at the same time as the amount of land required to produce the grain actually declined by approximately 56% (World Bank, 2008).
There is clear scope for potential increases in world food production on existing agriculture land, and these increases should be feasible utilising existing and newly developed technology. It has been estimated that in parts of Southeast Asia the average rice yields are only 60% of their average maximum climate-adjusted yields (Godfray et al. 2010). In addition, 11 countries are producing 37% of the total world wheat yield on predominantly rain-fed production conditions, well under their attainable yield potential (World Bank, 2008). If farmers around the world were able to produce closer to their potential yield, the world’s production levels should significantly increase without any additional land being brought into cultivation. This increase in production should equal about 23% of the current world production. As has been shown in the past 50 years, any local/national improvement in cultivars and management will spill over into the rest of the world. The world needs to understand that agriculture is not a local/national industry and what changes are made on a local/national level will have global impacts. On the other hand, the droughts in Australia and Argentina seem tied to global climate change, as does the irregularity of the monsoon season in India. However, food production increases will surely be subject to a number of additional constraints yet to be defined.
Even though this discussion indicates the potential for major increases in world agricultural production on existing cultivated land, based on existing and newly developed technology there are at least three impediments that could limit increased world food production. First, the technology and management improvements required for advancing yield levels might not be accessible or applicable to all crops, regions, and farmers of the world. Second, advanced technology and management inputs in agriculture could spread into areas of the world where they could accelerate environmental problems and have an adverse impact on biodiversity. Third, the public understanding of agriculture certainly needs to be vastly improved for productivity to be increased. Major yield increases will be based mainly on the improvement of productivity on land that is already being cultivated. To obtain the 8.22 BT of food produced in 2007 using 1963 cultivar/management technology, which generated a yield of roughly 2.63 BT in that year (UN-FAO FAOSTAT, 2010), would have required more than an additional 1.6 billion hectares of new land to be brought under cultivation, which would have had a profound detrimental impact on the world’s environment and biodiversity. Intensifying agricultural technology on existing lands, therefore, has had and will continue to have a major role in preserving biodiversity and maintaining the sustainability of our fragile global environment overall.
One vital need to eliminate hunger and poverty involves the preservation of sufficient genetic diversity in plants and their relatives to ensure that breeders have the capacity to create cultivars capable of resisting biotic and abiotic stresses and adapting to new environmental conditions. Existing and newly developed biotechnological tools alongside traditional technology will play major roles in improving world food production in the same manner as the ‘Green Revolution’ that occurred from the 1960s through the 1980s. This will amount to what Gordon Conway (1999) termed “the doubly green revolution.” The main problem will be the degree to which individual countries, industrialised and developing, can manage new technologies to adapt and improve their production with advantageous changes in agronomic practices and production costs without any adverse effects on the world’s environment and biodiversity.
The domestication and development of crops over the past 10 500 years has involved progeny selection from the most productive individuals based on their phenotypes. Modern biotechnology is taking plant improvement to new heights with the potential of greatly improving food quality and production (Federoff et al. 2010). New agricultural technologies are composed of individual systems that pivot around both public and private breeders’ plant selection programmes and involve many technologies including: first, tissue culture, in which plants can be broken down into cell suspensions that are manipulated, followed by regenerating plants, bypassing the traditional approaches to seed production. Second, the utilisation of anther culture coupled with various chromosome-doubling techniques, to successfully create double haploid populations, greatly reducing the time required to produce commercial cultivars. Third, modern approaches to mutation technology have been and continue to be successful in creating additional genetic variation necessary for crop improvement programmes. Fourth, and most recently, the utilisation of molecular marker-assisted selection, where various types of DNA marker systems are linked to traditionally difficult to screen value-added traits of interest that have already been successfully used in cultivar improvement programmes. Types of DNA marker system include; restriction fragment length polymorphism (RFLP) and amplified fragment length polymorphism (AFLP) (which at the present time are not being widely used for marker-assisted selection), simple sequence repeat or microsatellite repeat (SSR), single nucleotide polymorphism (SNP), diversity array technologies (DArT), etc. which are more commonly used (for an excellent review see Tester and Langridge 2010). Fifth, involves the application of genome-wide selection which is mainly used for selection involving quantitative traits in both animals and plants (Goddard and Hayes, 2009; Mayor and Bernardo, 2009). However, genome-wide selection requires the availability of a massive number of very cheaply available molecular markers (up to 20 markers per cM), or high-density marker chips designed specifically for the species being manipulated (which can be very expensive technology). Also, when selecting on a genome- wide scale, one has to consider the presence of considerable linkage drag or undesirable gene complexes linked to the value-added traits of interest. Sixth, the application of genomic sequencing of individual plant genomes to expose the location and potential function of the entire genetic composition of an organism which can be used in conjunction with other technologies to assist cultivar improvement programs. Seventh, plant transformation technology creates genetically modified organisms (GMOs), which involves transferring genes from one organism to another bypassing the sexual process, and has already been successful in several crops on a world scale.
Most of the traditional and newly developed technologies have been adapted to work efficiently in a more land- and labour-intensive form of agriculture improvement. It is clear that traditional organic farming applications are neither capable of producing enough to feed nor of improving world dietary standards of the existing population, let alone the projected increase to nine billion people by 2040. It has been estimated that a world population of approximately four billion people could be sustained if organic nitrogen farming systems were in place on a global scale (Buringh and van Heemst, 1977; Conner, 2008; Evans, 1998; Smil, 2001 and 2004), though requiring significantly more land under production to generate the required organic nutrients.
Discussion
Existing data indicate that agriculture is capable of feeding the projected world population increases on approximately the same amount of land that is currently under production (World Bank, 2008). However, it will take all of the available technologies and plant breeders’ skills to achieve the desired goal of eliminating hunger. In addition, integrated pest management, water management, precision farming, limiting chemical input and many other techniques, including the expansion of the infrastructure within many countries, must be wisely applied simultaneously to maximise yield from existing farmland. Only the coordinated application of all of these techniques will improve the productivity of the lands currently cultivated to the degree needed.
Significant progress has been made over the past several years in advancing our knowledge of biological science which can be applied to technology adaptable to improving agricultural production. We do not know the direction current research will take, but we can assume that any commercial application is going to be determined by world economic and social factors. The private sector and many funding agencies are increasingly addressing developing countries’ agricultural needs. The assertion that a “back to nature” or “pure organic” approach can feed the world’s people is only a theory that clearly does not take into account the current world population and the scale of human suffering from malnutrition and starvation. Embracing social justice is the only way that people can really survive and prosper, and we need to utilise all of our resources to accomplish the goal of feeding humanity.
All crops will continue to be improved by traditional and biotechnological approaches to advance their yield potential. Building adaptable gene complexes into crop varieties for the future is something that we must do, especially in the face of global climate change and the world’s increasing population. This process will require a much larger number of cultivars with different genetic backgrounds to be spread around the world than was the case in the past. In spreading new varieties, including transgenic crops, it is very important that we consider their potential impact (to the degree it is possible to predict an impact) on the world’s natural environment and biodiversity. Such concerns are being addressed, but as with most human endeavours, these issues must be dealt with on an individual crop and environmental basis. In future crop development several factors seem especially important. First, we need to understand the characterisation of the genome structure, gene function and regulation, and evolution at macro- and micro-geographic scales of all crops. Second, we need to combine single- and multi-gene value-added traits to produce a higher degree of cultivar development. Third, crop genetic systems must be analysed to determine the genetic flexibility of various species in diverse ecological contexts, according to their breeding systems, mutation rates, genome recombination properties, genomic distribution, and function of structural genes (primarily abiotic and biotic stress genes). Fourth, we need to characterise the interface between developing agricultural ecological dynamics and adaptive ecosystems in order to manipulate genome composition and limit the potential for gene contamination. In the past, when modern agriculture competed with the traditional subsistence forms of agriculture, local landrace cultivars were often discarded in favour of the new high yielding cultivars. Recently, massive efforts have been undertaken to preserve crop diversity, which has resulted in the retention of more old and new crop diversity of all kinds in agriculture than was retained 50 years ago. National and international seed banks are, and will continue to be, critically important to agriculture and the maintenance of the world’s biodiversity.
Conclusions
The continued long-term health of world food production is one of the foundations of world security. A stable future for humanity, our environment, and our biodiversity is intimately tied to the improvement of crop production.Adequately feeding the world’s population is clearly one of the most important challenges facing the world today and in the future. Existing data indicate that agriculture is capable of feeding the projected increased world population on approximately the same area of land as that currently under production (World Bank, 2008). However, it will take all the available, relevant and reliable technologies, and the unwavering dedication of all involved to achieve the desired goal of eliminating hunger.
References
Barrett, C.B. (2010) Measuring food insecurity. Science, 327, 825-828.
Buringh, P., & van Heemst, H.D.J. An estimation of world food production based on labour-oriented agriculture. Centre for World Food Market Research, Amsterdam. 1977.
Bruinsma, J. (ed.) World Agriculture: Towards 2015-2030 An FAO Perspective. London, Earthscan Publications Ltd, 2003. I Conner, D.J. (2008) Organic agriculture cannot feed the world. Field Crops Res., 106, 187-190.
Conway, G. The doubly green revolu- tion: food for all in the twenty-first centu- ry. Ithaca, Cornell University Press, 1999. I Evans, L.T. Feeding the Ten Billon.
Plants and population growth. Cambridge, Cambridge University Press, 1998.
Federoff, N.V., Battisti, D.S., Beachy, R.N., Cooper, P.J.M., Flischhoff, D.A., Hodges, C.N., Knauf, V.C., Lobell, D., Mazur, B.J., Molden, D., Reynolds, M.P., Ronald, P.C., Rosegrant, M.W., Sanchez, P.A., Vonshak, A., & Zhu, J.-K. (2010) Radically rethinking agriculture for the 21st century. Science, 327, 833-834. Godfray, H.C., Beddington, J.R., Crute, I.R., Haddad, L., Lawrence, D., Muir, J.F., Pretty, J., Robinson, S., Thomas, S.M., & Toulmin, C. (2010) Food security: The challenge of feeding 9 billion people. Science, 327, 812-818.
Goddard, M.E., & Hayes, S.J. (2009) Mapping genes for complex traits in domestic animals and their use in breeding programmes. Nature Reviews Genetics, 10, 381-391.
Mann, C.C. (1999) Future Food: Crop Scientists Seek a New Revolution. Science, 283, 310-314.
Mayor, P.J., & Bernardo, R. (2009) Genomewide selection and marker-assist- ed recurrent selection in doubled haploid versus F2 populations. Crop Science, 49, 1719-1725.
Pingali, P.L., & Rosegrant, M.W. (1998) Supplying wheat for Asia’s increasingly westernized diets. American Journal of Agricultural Economics, 80, 954-959.
Smil, V. Feeding the world: A challenge for the twenty-first century. MIT Press, Cambridge, 2001.
Smil, V. Enriching the earth: Fritz Haber, Carl Bosch, and the transformation of world food production.Cambridge, MIT Press, 2004.
Tester, M., & Langridge, P. (2010) Breeding Technologies to Increase Crop Production in a Changing World. Science, 327, 818-822.The United Nations Food and Agriculture Organization FAOSTAT. (2010) (http://faostat.fao.org/site/368/Desktop Default.aspx?PageID=368#ancor)
The United Nations Food and Agriculture Organization FAOSTAT. (2010) (http://faostat.fao.org/site/550/Desktop Default.aspx?PageID=550#ancor)
The United States Department of Agriculture-National Agriculture Statistics Service (USDA-NASS) (2010) http://www.nass.usda.gov/World Bank. World Development Report: Agriculture for Development. Washington, D.C., The World Bank, 2008.
Download pdf