The functions and sizes of the five carbon sinks on planet Earth and their relation to climate change Part 2 Coniferous forests-do they warm or cool the climate?
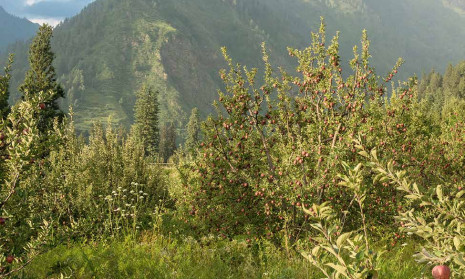
Summary
The UK Government and Forestry Commission state: “if we plant an additional 15,000 hectares per year, by 2050 these woodlands could be sequestering carbon equivalent to 10% of GHG emissions”(1)(Appendix A).
The inference is that by lowering atmospheric CO2 concentration with planting conifers climate cooling will follow. This is not so. This premise is wrong.
Latitude-specific deforestation experiments indicate that afforestation projects in the tropics would be clearly beneficial in mitigating global-scale warming, but would be counterproductive if implemented at high latitudes (50-70N) and would offer only marginal benefits in temperate regions (40-60N). Although these results question the efficacy of mid- and high-latitude afforestation projects for climate mitigation, forests remain environmentally valuable resources for many reasons unrelated to climate.(2)
GHGs produce their effects by increasing the retention of solar short wave radiation (lowering the albedo index (0 to 1) and by increasing radiative forcing measured in Watts per m-2, compared with the effects of an atmosphere of oxygen and nitrogen.
Forests themselves and grassland or cropped land also absorb short wave radiation, but coniferous forests do so to a greater extent than does grassland, or field crops.
Hence these forests increase radiative forcing – i.e. forestation, especially between the latitudes of 50 to 70 (virtually none in the southern hemisphere is at >50 S) are liable to do so to an extent that exceeds the rate of their C sequestration in these colder latitudes, where there is less solar radiation. Moreover, as forest C sequestration reduces atmospheric CO2 at a rate that is a lower proportion of the amount sequestered, it is less effective than might be expected.
This effect owes to a compensating release of CO2, especially from oceans but also from other vegetation (feedback). The net effect is positive for radiative forcing (Appendix A) for at least 90 % of UK coniferous forests. Forests may also increase water vapour in the atmosphere and so promote cloud formation - a useful function in arid climates.
The influence of water vapour is likely to be variable and, although probably positive for radiative forcing, it is not accommodated in the calculations here, owing to a lack of reliable data. All people enjoy seeing trees.
Nevertheless, we contend, especially in the present era with a rising world population and a scarcity of free land space, that afforestation should be carried out for the appropriate reasons, based upon reliable evidence. In the next Issue we shall describe how we consider this anomaly should be addressed and corrected.
This will include a discussion of the life expectancy and effects of the cut timber which have not so far been considered by the protagonists of this subject.
Glossary
Albedo is the "whiteness" of a surface. Albedo is the ratio of solar radiation reflected from the surface to the incident radiation. It is a dimensionless coefficient on a scale from zero (no reflection) of a black surface to 1 for perfect reflection of a white surface.
Boreal forest (the taiga, Russian, a swampy forest) occurs throughout high northern latitudes, from about 50°N to 70°N, between the tundra, and the temperate forest, with average annual temperatures of about +5° to -5° C.
It is bordered to the north by treeless arctic tundra and to the south by temperate forest or grassland. The northern woodland is of widely spaced coniferous trees, hardy low shrubs, and lichens.
The main boreal forest is closely spaced evergreen and deciduous trees, as well as shade-tolerant shrubs with herbs and moss. The southern region contains occasional temperate deciduous trees. Evergreens: black and white spruce, jack and lodge pole pine, and balsam fir, American larch, birch, aspen, and balsam poplar.
Carbon offset is a reduction in emissions of CO2, or other greenhouse gases (GHGs) made in order to compensate for, or to offset, an emission made elsewhere. It is measured in metric tonnes of carbon dioxide-equivalent (CO2e).
CCCarbon-cycle Feedbacks, see Appendices B and C
Latent heat is the energy released or absorbed, by a body, or an isolated system, during the conversion of a solid into a liquid or vapour, and of a liquid into a vapour, or the reverse of these processes, at a constant temperature. In this text it relates to the evaporation of water transpired by trees and its subsequent condensation in cloud formation, returning to earth as rain (Appendix D).
Latitudes In the Northern Hemisphere high latitudes are 50°N to 90°N, mid-latitudes, 20°N to 50°N, and tropics, 20°S to 20°N. Temperate zones include, approximately 40-60 N and in the southern hemisphere temp. zone: New Zealand, Tasmania and southern tip of S. America, including much of Tierra Del Fuego, 40-50 S.
Radiative forcing or climate forcing is the difference of insolation (sunlight) absorbed by the Earth and that radiated back to space. It is quantified in Watts per square metre of the Earth's surface (Wm-2). The Watt the SI unit of power, equivalent to one Joule (J) per second. A positive forcing (more incoming energy) warms the system, while negative forcing (more outgoing energy) cools it. (1 Watt, W = kg.m-2s-3 = 1 J.s-1 of energy). The debate on global warming can be described and quantified in an equation of radiative forcing, excluding the important specific effects of, for example of CO2 in ocean acidification not directly connected to climate warming.
Abbreviations:
K (temperature) KELVIN, CELSIUS
Water boils, 373.16K, 100°C
Water freezes, 273.16K, 0°C
Absolute zero, 0.00K, -273.16°C
PAR, Photosynthetically active radiation, measured as, J m-2 s-1,or, Wm-2
Petagram, Pg, 1015 g Picograms, pg, 10−12 g
Rs Respiration rate –used here to describe the O2 consumption by trees, or soil microorganisms and the production and release of CO2.
Introduction
The target of the UK Government’s carbon budgets(1) include a reduction of 34% in GHG (Greenhouse Gas) emissions by 2020, based on 1990 levels. There is a longer term commitment for an 80% reduction of GHG emissions by 2050. “Ideally these changes will be made by reducing direct emissions of CO2 and other greenhouse gases.
It is estimated that if we plant an additional 15,000 hectares per year then by 2050 these woodlands, could be sequestering CO2 equivalent to 10% of GHG emissions by 2050”.(1)
The Intergovernmental Panel on Climate Change(3) concluded that "a sustainable forest management strategy aimed at maintaining or increasing forest carbon stocks, while producing an annual sustained yield of timber fibre or energy from the forest, will generate the largest sustained mitigation benefit.
Sustainable management practices keep forests growing at a higher rate over a potentially longer period of time, thus providing net sequestration benefits in addition to those of unmanaged forests”(3).
Forest systems cover more than 4.1 x 109 hectares of the Earth's land area (4,5) with a biomass of 56-165 t C ha-1 and total organic matter double this value(6); two thirds of this will be in the underlying soil(4).
A value of 400 x 109t.C is a fair estimate of the total global living and dead forest organic matter, above plus below the soils surface. An estimate three times as large(7,8) is given in Appendix C (also see Table 1).
As temperate forest represent only 14% of total forest C, can their replanting be justified as a means of increasing worldwide C sequestration? Must their justification be on different grounds?
These values compare poorly with the annual global anthropogenic CO2 emissions from fossil fuel and cement manufacture of 9-10 x109 t C an.-1 (9).
None of the statements in Appendix C includes measures of radiative forcing, or values of albedo for darker coniferous forests versus those for grassland (see Appendices E and F).
A change in land use from pasture to coniferous forest results in a decrease in albedo, which has a direct effect on the surface radiation balance (10). The increase in absorbed solar energy leads to an increase in radiative forcing, resulting in warming of the planet. In addition, land-use change can have indirect effects through changes in evaporation that subsequently affect cloud formation, affecting both short- and long-wave components (2,11) (also see Appendix D).
In this article the likely quantitative role of temperate coniferous agroforestry as a source of environmental services, especially that of C sequestration and the potential of a UK coniferous forest for countering global warming will be estimated.
A comparison will be made of the rate of carbon storage in conifers with that of the difference in albedo between those trees and pasture, as an alternative use of that land, using the same terms for energy, Watts and Joules. The conclusion will advanced that excessive use is made of coniferous forests in the UK.
In temperate regions as exemplified by forestation in the UK (latitude 50 -60 N) it has been stated that “Over the long-term average, taking into account the whole cycle of a commercial rotation, the sequestration rate can be as high as 3 t C per hectare per year”, but an average rate of 2.5 t Cha-1yr-1. with harvesting at 100 t Cha-1 after 40 years is standard for the UK Forestry Commission (1) (Appendix A).
The effect of albedo and radiative forcing (RF) in the temperate regions of the World Atmospheric carbon dioxide has the effect of retaining short wave solar radiation – a necessary function when its concentration is within the range of 380-390 ppm, to ensure the biosphere is held within a range of temperatures which sustain life. In its absence the surface of the Earth would be very cold.
The atmospheric CO2 therefore has a high RF value.
Global warming is caused by the Earth’s net RF of solar energy received by the Earth’s surface increasing(3,5,7,10) with CO2 and other GHGs being one group of contributors to this.
The other major factor is the reflective properties of the surface of the Earth. Kirschbaum et al.(11) measured the RF value of a coniferous forest in New Zealand and found for a given level of solar radiation coniferous forests have a relatively low albedo and so their RF value is relatively high in comparison to grassland, or other crops.
This poses an important question: Is the rate of carbon accumulation during tree growth, per ha, both above and below ground, measured in terms of RF, as the atmospheric CO2 it replaces, greater than the difference in RF between the forest and the alternative land cover, also measured in this common denominator? One should accommodate other sources of GHG, especially those of anthropogenic origin on grass/crop land, or in cultivated forest and differential effects on soil microorganisms (see next Issue of WA).
Moreover, evapo-transpiration by forests tends to stimulate cloud formation, which can be valuable in arid regions, but will also influence climate (Appendix D). The energy used in transpiration and the latent heat (see Glossary and Appendix D) of evaporation of moisture from trees and subsequent condensation in cloud formation can be considered a closed thermodynamic system in which there is no, or little, effect on temperature. Nevertheless, the increase in the atmospheric concentration of invisible moisture represents a potent GHG (Table 2 and Appendix D).
It should also be noted that if the comparison was only between forest C sequestration and fossil fuel consumption, both would affect feedback. Feedback is not usually measured in discussions of fossil fuels, so should not be used in estimating the C sequestration for that comparison.
However, albedo differences influence world temperature directly, without influence on atmospheric CO2, so it is relevant to include an estimate of Feedback in the comparison of C sequestration with albedo differences of land use to be discussed here (feedback, see Glossary and Appendix B).
Presently tropical and subtropical forests are being destroyed and temporal and boreal forests are being retained, or increased. There is a different rate of growth and of C accumulation in the various regions of the world.
The area of mid-high latitude forest is assumed to cover 2.5 x 109 hectares – an area greater than that of tropical rain forest (2 x 109 ha). But the latter could accumulate double the annual rate of C sequestration (Appendix C)(5,6) of those at high latitudes. Thus, the regions of the planet in which forests grow could be critical to the control of global warming.
Betts(10) concluded that in many boreal forest areas, the positive forcing induced by decreases in albedo is likely to offset the negative forcing that is expected from carbon sequestration. Some high-latitude forestation activities may therefore increase climate change, rather than mitigating it, as intended.
Several groups of workers have concluded (2,5,10) that global-scale deforestation has a net cooling influence on Earth’s climate, because the warming carbon-cycle effects of deforestation are overwhelmed by the net cooling associated with changes in albedo and evapotranspiration from forest canopies.
The replacement of high latitude forests by grasses and shrubs increases the surface albedo by as much as 0.25. This results in decreased absorption of surface solar radiation and cooling that exceeds 6 K in some locations, despite higher CO2 concentrations and high-latitude amplification of CO2-induced warming.
The albedo effect therefore dominates the climate response in the Northern Hemisphere mid- and high latitudes (2,11,12). Moreover, the fourth IPCC Assessment report (13) summarised studies that had estimated the change in surface albedo with the expansion of agriculture since pre-industrial times.
It concluded that land-use change is likely to have led to a radiative forcing of −0.2 ± 0.2 W m−2, leading to a global change of about −0.1◦. Nevertheless, it is not being suggested here that boreal forests should be destroyed.
Estimating the radiative forcing of a temperate coniferous forest
How is forest to be valued as a means of helping to curtail the rate at which climate is changing?
Re-release (feedback) of atmospheric CO2 is approximately one third of the amount sequestered(11). The radiative force equivalent to two thirds of the sequestered C should exceed the direct increase in the radiative forcing caused by the forest less that caused by alternative land use of that area.
In order to calculate this it is necessary to know the energy equivalent as radiative forcing in watts (W) per square metre of land surface (W m−2) per unit of CO2. This is 5.35 W m−2(11). Units of CO2 are converted to radiative forcing using this factor(3). The atmospheric gas is reasonably uniformly distributed over the earth so it is necessary to make our calculation worldwide per year to allow for seasonal variation.
A change in forest carbon stocks, ∆C [tC], needs to be converted to the corresponding concentration change in the atmosphere, ∆[C]. One ppm (molesx106/mole) of CO2 concentration in the atmosphere corresponds to 2.123 GtC (14).
As watts are a rate it is more useful to convert the energy to Joules. Using 2.123 GtC and 5.35 Js-1m-2 (in place of 5.35Wm−2) the resultant value for the removal from the atmosphere of 1 ppm CO2 would be a change in radiative forcing, (∆RE), over a year for the Earth as a whole by −104 GJ tC−1 yr−1(11).
This value makes no allowance for feedback. For the application of these data to New Zealand and to NE China see Appendix E and Fig.1.
Under New Zealand conditions, it could be concluded that the albedo changes negated the benefit from carbon storage over a rotation of a conifer forest by 17 or 24%, depending on the omission or inclusion of the carbon-cycle feedback.
Thus, by the end of the rotation, the carbon cycle feedback reduced the beneficial effect of carbon storage in trees by about one third (Fig. 2). The radiative forcing changes resulting from albedo changes only diminished but did not negate the climate-change benefit from tree plantings and tree planting at Puruki (latitude 38◦2514411 S) is concluded to be worthwhile from the viewpoint of promoting global cooling on good quality soil with relatively high rainfall.
Betts (2000)(10) assumed carbon storage potentials between about 100 tC ha−1 for cold or dry regions in Siberia, Canada or China and 200 tC ha−1in more temperate regions of Europe and North America. The Puruki stand reached carbon storage of 100 tC ha−1 after about 9 years and 200 tC ha−1 after 17 years (Fig. 1).
Conclusions over Radiative Forcing of UK Confinerous Forests
The purpose of this review was to determine whether C sequestration of coniferous forests in the UK, for which the UK Forestry Commission (FC) has published values(1), is at a rate which benefits or retards global cooling.
Using the FC values the albedo and consequential RF for UK coniferous forests were estimated relative to those for grassland and agricultural crops which might occupy the same land area. The data sets were compared in standard energy terms.
Reliable data for the UK are scarce, so in this paper global published data, together with data from NZ also been used conservatively. It has been assumed that solar radiation averages 13.5 MJ m−2 d −1 and that there is a mean albedo difference between the two land use types of 9.5 %. Nevertheless, because of the uncertainty of these norms a range of values has been tested.
Higher values than these would certainly confirm the thesis. Lower values were tested down to 11.5 MJ m−2 d −1 for solar radiation and to 8.0 % as mean albedo difference. Summary equations developed are given in Appendix E. Although many equations were tested, only those describing the extremes are given.
These show that under a wide range of possible values for solar radiation and albedo difference, it is unlikely that coniferous forests in the UK would benefit global cooling, but rather, they are more likely to warm the climate, compared to the effects of alternative crops.
It would have taken 21 years for the forest to become neutral (c.f. 4.5 yr in NZ), or it would have been necessary that 5.6 tCha-1yr-1 were accumulated for the rate of growth to achieve neutrality in terms of its effect on climate.
This conclusion is certainly true where feedback is included. What is surprising is that it is also the case if feedback is ignored! Irrespective of the quantitative importance of albedo in specific regions and circumstance, the Puruki and previous studies (2, 10, 11,18) showed that albedo changes can be important enough to be considered in an overall assessment of the radiative forcing consequences of a change in land use (14).
Land-use and land-cover changes (LULCCs) are known by recent studies (19) to influence climate directly, by altering surface physical properties such as albedo, or water transpired from soils to the atmosphere. These effects are most pronounced at regional scales, but some predictions of future LULCC affect global mean quantities, such as temperature and precipitation.
The conversion of 1 km2 of woody vegetation (forest and shrub land) to non-woody vegetation (crops and grassland) yielded between 0 and −0.71 nW/m2 of globally averaged radiative forcing determined by the vegetation characteristics, snow dynamics, and atmospheric radiation environmental characteristic within each of the 151 global regions(19).
Across a set of scenarios designed to span a range of potential future LULCC, the forcing ranged from −0.06 to −0.29 W/m2 (Fig. 3) by 2070, depending on assumptions of future crop yield growth and whether climate policy favoured afforestation or bioenergy crops.
“This range is similar in magnitude to historic forcing from several factors, including the GHG N2O, nitrous oxide. Inclusion of this previously uncounted forcing in the policy targets driving future climate mitigation efforts led to changes in fossil fuel emissions of 1.5 PgC/yr by 2070, corresponding to a 12–67% change in fossil fuel emissions depending on the scenario” (19).
It is accepted that this analysis purposely ignores several aspects of the need to control GHGs – e.g. the effect of increased atmospheric CO2 on ocean acidification.
Nevertheless, climate change cannot be mitigated efficiently if management response strategies consider only some radiatively important factors (e.g. carbon storage) while ignoring others (e.g. direct effects on the Earth’s energy balance).
Optimal management of the Earth’s biosphere for climate-change mitigation can only be achieved if all relevant factors are considered, quantified and included in an overall assessment.
Conclusions
Change to the Earth's albedo is a powerful driver of climate. When the planet's albedo or reflectivity increases, more incoming sunlight is reflected back into space. This has a cooling effect on global temperatures. Conversely, a drop in albedo warms the planet.
A change of just 1% to the Earth's albedo has been estimated to have a radiative effect of 3.4-3.7 Wm-2, comparable to the forcing from a near doubling of CO2.(20)
Increased carbon storage with afforestation leads to a decrease in atmospheric CO2 concentration and thus decreases radiative forcing which cools the Earth. However, afforestation also changes the reflective properties of the surface vegetation from more reflective pasture to relatively less reflective forest cover. This increase in radiation absorption by the forest constitutes an increase in radiative forcing, with a warming effect.
The net effect of carbon storage and of decreased albedo on radiative forcing depends on the relative magnitude of these two opposing processes.
Deforestation has a net cooling influence on Earth's climate, because the warming carbon-cycle effects of deforestation are overwhelmed by the net cooling associated with changes in albedo and evapotranspiration. The global-mean temperature differences relative to the Standard case in year 2100 in the Tropical, Temperate, and Boreal experiments are +0.7 K, −0.04 K, and −0.8 K, respectively (2), indicating the value, in climatic terms, of retaining tropical forest.
Our calculations for the UK (latitudes 49.4N-60.5N) indicate that coniferous forestry warms the climate relative to the effects of agricultural crops. Less than 10% of such forests in the UK would have a climate cooling effect. The explanation is that the growth rate of trees and therefore the C-cycling effect is over-whelmed by the biophysical effects of radiative forcing relative to grassland and other agricultural crops.
It has been concluded that the indirect effect can be of a similar magnitude (and opposite sign) as the direct, ground-based albedo changes, especially in more tropical locations. But as atmospheric invisible moisture is a potent GHG, the balance is positive for radiative forcing in temperate and boreal regions for the effects of atmospheric moisture and albedo of forests (Falloon et al. 2012)(21), see Appendix F).
Latitude-specific deforestation experiments indicate that afforestation projects in the tropics would be clearly beneficial in mitigating global-scale warming, but would be counterproductive if implemented at high latitudes and would offer only marginal benefits in temperate regions.
It has been concluded that tree growth rate of boreal forests and possibly at least some temperate forests, between latitudes 50 N and 70 N are of questionable value as carbon sinks. Their justification should be on other bases - e.g. where appropriate to reduce flooding and ocean acidification, increase biological diversity and as an amenity or as a source of building material and fuel. If a forest between these latitudes fails to meet any of these objectives then its value should be questioned, especially if cropland is used for urban building purposes instead of planted coniferous forest.
It is accepted that this analysis purposely ignores several aspects of the need to control GHGs- e.g. the effect of increased atmospheric CO2 on ocean acidification. Moreover, forests remain environmentally valuable resources for many reasons unrelated to climate, but large-scale afforestation implemented in temperate latitudes may be largely ineffectual in mitigating global warming and could compete with areas necessary for food production, as world population increases and tropical areas become too hot for crop production.
Hence rational policy decisions must be taken only with possession of all relevant established facts. The purpose of this review has been to establish those facts concerning mitigation of climate change. Climate change cannot be mitigated efficiently if management response strategies consider only some radiatively important factors (e.g. carbon storage) while ignoring others (e.g. direct effects on the Earth’s energy balance). Optimal management of the Earth’s biosphere for climate-change mitigation can only be achieved if all relevant factors are considered, quantified and included in an overall assessment.
APPENDICES
Appendix A
UK Forestry Commission
Climate Change and British Woodland JUNE 2005
“UK Government, Forestry Commission (1) targets presented in the UK’s carbon budgets are to reduce GHG emissions 34% by 2020, based on 1990 levels. There is a longer term commitment to reduce GHG (Greenhouse Gas) emissions 80% by 2050, based on 1990 levels. Ideally these changes will be made by reducing our direct emissions of CO2 and other greenhouse gases. It is estimated that if we plant an additional 15,000 hectares per year on top of the current planting rate of 8,000 hectares per year, then by 2050 these woodlands, together with those already planted since 1990, could be sequestering an amount of CO2 equivalent to 10% of GHG emissions, assuming the target above is met. So if we plant trees now, they will be compensating for a small but significant proportion of our emissions by 2050.”
Appendiix B: FEEDBACK
The carbon (C) stored in trees lowers the atmospheric carbon dioxide concentration, which in turn reduces the carbon dioxide up-take by the natural global C pools, especially the oceans), as a fraction of C is exported from the oceans to the atmosphere.
The atmospheric carbon dioxide concentration is therefore reduced by approximately one third less than the amount of C stored in trees from the evidence of Kirschbaum, et al. (11), but the difference increases over the time of carbon storage.
To calculate the benefit of saving the emission of 1 tonne of fossil fuels against the benefit of storing 1 tonne of C in the biosphere, the comparison therefore needs to be consistent in either including or excluding C cycle feedbacks in both instances. As these feedbacks are not generally included in calculating the effect of fossil-fuel management, it would be appropriate to base comparison on stand-level calculations.
On the other hand, comparisons of C sequestration by forests with the albedo difference between forest and grass, or other crops, it is relevant to include feedback effects of C sequestration, as atmospheric CO2 is not involved in the measurement of albedo differences.
Appendix C
Global forest Carbon
Globally, forest vegetation and soils contain about 1146 petagrams, Pg, (1146 x 109t C) of carbon in forest vegetation and soils, with approximately 37% of this carbon in low-latitude forests, 14% in mid-latitudes, and 49% at high latitudes (7) (Table 1). In 1990, deforestation in the low latitudes emitted 1.6 +/- 0.4 petagrams (1.6 +/- 0.4 t C x 109) of carbon per year, whereas forest area expansion and growth in mid- and high-latitude forest sequestered 0.7 +/- 0.2 petagrams of carbon per year, for a net flux to the atmosphere of 0.9 +/- 0.4 petagrams of carbon per year (5).
Geographically, approximately 470 Pg C (55%) is stored in tropical forests, 270 Pg C (32%) in boreal, and 120 Pg C (14%) is in temperate forests. The C stock density in tropical and boreal forests is comparable (242 versus 239 Mg C ha–1), whereas the density in temperate forests is ~60% of the other two biomes (155 Mg C ha–1). Afforestation and reforestation potentially could achieve annual carbon sequestration rates in above ground and below-ground biomass of 0.4-1.2 t ha–1 yr–1 in boreal regions, 1.5-4.5 t ha–1 yr–1 in temperate regions, and 4-8 t ha–1 yr–1 in tropical regions (5).
Although tropical and boreal forests store the more carbon, there is a fundamental difference in their carbon structures: Tropical forests have 56% of carbon stored in biomass and 32% in soil, whereas boreal forests have only 20% in biomass and 60% in soil (8).
Hence, worldwide there is a net loss nearly 1 x 109 t C an.–1 from forests, owing to removal of tropical forests and the planting of temperate forests. This represents an annual loss of between 0.1 and 0.25% of the total forest C, both above and below ground. Nevertheless, temperate forests contain only 60-65% of the Cha–1 of the density in tropical and boreal forests.
Appendix D
Clouds, Evapotranspiration and water vapour
Latent heat of transpired water
It is the heat required to convert a solid into a liquid or vapour, or a liquid into a vapour, without change of temperature. It is energy released or absorbed, by a body or a thermodynamic system, at a constant-temperature and pressure. An increasing amount of water vapour in the atmosphere absorbs some outgoing long-wave radiation, prevents it from escaping from the Earth system and adds to global warming.
As the water vapour cools and condenses to form clouds, it releases this latent heat into the atmosphere, where it sheds the heat as infrared radiation. Water vapour and clouds, once again, contribute to Earth's energy budget and hence its climate balance.
Clouds prevent some outgoing long-wave radiation escaping from the Earth, but low clouds tend to have high albedo, so they predominantly reduce the short-wave flux to the surface and thus cool the Earth. The resultant radiation balance is complicated by the changes in the partitioning of available energy into latent heat and sensible heat.
About one sixth (17%) of incoming sunlight is reflected back into space by clouds. Water vapour and clouds combine to "intercept" 59% to 76% of the outgoing infrared radiation.
Changes to the amount of water vapour of the number of clouds seem certain to have an important effect on climate. However, water vapour and clouds play numerous roles in the climate system, and the net effect of increased evaporation rates caused by global warming are difficult to predict.
Forests can be cooler than neighbouring pasture, primarily because of the effects of evaporation, which cools the local environment. However, that local cooling is not relevant in the global context as evaporation simply transfers heat from the evaporating surface to the atmosphere. When the water vapour condenses as liquid in clouds or rain, the heat is returned to the atmosphere. On the global scale, the effects of evaporation and condensation cancel each other out with no net effect. Only effects on the radiative balance remain.
Appendix E
Conversion of micromoles CO2 per second to mass of C per year:
Micromoles CO2 m-2 s–1 are converted to g C m-2 yr-1 by using the molar mass of C: 12g/mol.
So it is: μmoles 12x10-6 x3600x24x365 = g C m-2 y–1.
And: t Cy–1 ha–1 = 12 x 10-6 x 3600 x24 x365 x 104 x 10-6;
n.b. (g C m-2 y–1= 378.43 x Micromoles CO2 m-2 s-1).
New Zealand (NZ) has a mean annual incident solar radiation of 13.5 MJ m−2 d–1 at Latitudes 35-47S (South Island av. rainfall = 178 cm, 70 inches/an., 16 rainy days/mn., solar rad.11-12 MJ m−2 d −1. North Island, rain 66 cm, 26 inches/an., 12-13 rainy days/mn., solar rad. 15 MJ m−2 d –1).
Average annual incoming radiation changes with latitude and with the extent of cloudiness (17). Kirschbaum et al. (11) used the mean annual incident solar radiation value of 13.5 MJ m−2 d−1 and an albedo difference between two land-use types of 7% (i.e. albedo values of 13% for a forest of Pinus radiate on good soil and 20% for pasture at Puruki in NZ, Fig. 1b) and from this formulated the following for annual radiative forcing, Ryr, of:
Ryr = 13.5 MJ m−2 d−1× 0.07 × (1 − 0.2) × 10 000 m2 ha-1 × 365 d yr-1 =
+2759 GJ ha−1yr−1. i.e. the mean difference in rate of incident solar radiation for the Puruki stand and grassland.
This value is the direct radiative forcing of the albedo difference per unit area per annum.
With an atmospheric carbon dioxide concentration of 390 ppm, and for the Earth as a whole, the radiative forcing, RE, of the removal of 1 tonne carbon =−104 GJ tC−1yr−1(11).
Then, ∆Ryr/∆RE =(2759 GJ ha–1 yr–1/−104 GJ tC–1yr–1) = -26. 5 tC ha–1. must be stored in a growing forest to balance the increase in radiative forcing resulting from the observed albedo change at Puruki in NZ. This stand reached a carbon storage of 26.5 tC/ha at a rate of 5.9 tC/ha/yr after 4.5 yrs (or 7.5 yrs, including feedback effects),100 tC ha–1 at a rate of 11.1tC/ha/yr after 9 years, but over the last 8.4years it accumulated 11.9 tC/ha/yr at a constant rate/yr to reach 200 tC ha–1. The overall at a rate of 11.5 tC/ha/yr for the total of 17.4 yrs (Fig. 1).
North Eastern China (Lat.40o-51oN) reported(16) that the surface net radiation of cropland is 7.53 W∙m−2 less in winter and 11.2 W∙m−2 less in summer than is that of forest. Moreover, the latent heat flux through evapotranspiration over cropland is less than that over forest, especially in summer (−19.12 W∙m−2). Average sensible heat flux increases in summer (7.92 W∙m−2) and decreases in winter (−8.17 W∙m−2), indicating that conversion of forest to cropland may lead to warming in summer and cooling in winter in NE China(15).
UK Data:
The Forestry Commission data indicate that planted coniferous forests in the UK and in other areas of these latitudes are harvested after they reach carbon storage of 100 tC ha–1 at about 40 years with approximately linear rate of growth. Based upon a similar pattern of growth to that in NZ the rate of growth in the UK would be 2.41 tC/ha.yr for years 0 to 20 and 2.59 tC/ha.yr for years 20 to 40 for the declared overall rate of 2.5 tC/ha.yr.
Moreover, for the UK at L 50-60 N Breuer et al. (2003)(22) summarised albedo values from a large number of authors and found albedos for coniferous forests to be an average of 12.5% and grassland albedo to average 23% (difference 10.5%).
Hollinger et al. (2010)(23) reported grassland albedos average 19.5% and 11% (difference 8.5 %) for evergreen needle-leaf forests in temperate locations), so it is assumed a conservative estimate of 9.5% for the grassland-forest difference with a mean annual incident solar radiation of 13.5 MJ m−2 d−1 in the baseline calculations for the UK.
Now radiative forcing, RE, led to a value of 26.5 tC ha-1 (see above) was required to be removed from the forest before the forcing is equalled under NZ conditions. But under UK conditions the baseline calculations would be:
∆Ryr = 13.5 × 0.095 × (1 − 0.2) × 10 000 × 365 = 3745 GJ ha–1 yr-1 , so 3745/-104 = -36 tC.ha–1
is the equivalent amount of forest carbon in the UK to the difference in albedo between coniferous forest and grassland over one ha/an. Alternatively, if we assume even lower solar radiations of 13.0 down to 11.5 MJ m−2 d –1 and an albedo difference between two land use types of 9.5% down to 8.0% then an annual radiative forcing difference between the coniferous forest and grassland would be the equivalent of:
∆Ryr = 13.0×0.095×(1−0.2)×10 000×365 = 3606.2 GJha–1yr-1 and 3606.2/-104 =-34.68 tC.ha-1.
∆Ryr = 11.5×0.095×(1−0.2)×10 000×365 = 3190.1 GJha-1yr-1 and 3190.1/-104 =-30.67 tC.ha-1.
∆Ryr = 12.0×0.08×(1− 0.2)×10 000×365 = 2803.2 GJha–1yr–1 and 2803.2/-104 =-26.95 tC.ha–1.
Thus, at least 26.9 tonnes of C per ha. needs to be removed from the forest/ha before cooling outweighs warming.
For the lowest likely albedo effect in the UK: 2.41 tC.ha–1yr–1 0.66 x Yyr = 26.95tC.ha–1, Y = 16.94 yr and excluding feedback: 2.41 tC.ha–1yr–1x Yyr = 26.95tC.ha–1 , so Y = 11.18 yr
With this small difference in albedo between forest and grassland producing the equivalent of 26.95 tC.ha–1 it would still take 11.2 years of forest growth, excluding feedback, to equal the albedo difference and nearly 17 years including feedback.
For the most likely situation in the UK 2.41 tC.ha–1yr–1 0.66 x Yyr = 34.68tC.ha–1 = 21.8 yr
2.5 tC.ha–1yr–1 0.66 x Yyr = 34.68tC.ha–1 = 21.0 yr
UK for 10 yrs would require YtC.ha–1yr–1 = 34.68 tC.ha-110 -1 0.66-1 = 5.63tC.ha–1yr–1 with feedback
Without feedback for 10 yrs it requires 3.714tCha-1yr–1
Least likely in the UK for 10 yrs YtC.ha–1yr–1 = 26.95tC.ha-110-1yr 0.66-1 = 4.083tC.ha–1yr–1 with feedback.
Least likely in the UK without feedback for 10 yrs it requires 2.7tcha–1yr–1 excl. feedback.
Appendix F:
Global temperature changes owing to interactive vegetation dominated by increases in shrub and needle-leaf trees at high latitudes and losses of broadleaf trees and grasses across the Amazon of + 0.43 and + 0.28 K per decade, respectively under a future system in which the world does not rely too heavily on one energy source and that similar improvement rates apply to all energy supply technologies and a climate stabilisation scenario.
In boreal (50-70 N) and temperate (23.5-66.5 N) regions the warming impact of reduced albedo (particularly during December–February and March–May) due to increased vegetation cover appears to have offset any cooling owing to the small evaporation rates. (Falloon et al. 2012)(21).
References
1 UK Gov’t Forestry Commission (2005) Climate Change and British Woodland, JUNE.
2 .Bala,G.,Caldeira,K, Wickett,M., Phillips,T.J, Lobell,D.B., Delire,C. and Mirin,A. (2007) Combined climate and carbon-cycle effects of large-scale deforestation. Proc. National Academy of Sciences, · vol. 104 no. 16, 6550–6555.
3 Ramaswamy, V., Boucher, O., Haigh, J. D., Hauglustaine, D., Haywood, J., Myhre, G., Nakajima, T., Shi, G. Y., and Solomon, (2001) S.: Radiative Forcing of Climate Change, in: Climate Change 2001: The Scientific Basis. Contribution of Working Group I to the Third Assessment Report of the Intergovernmental Panel on Climate Change, edited by: Houghton, J. T., Ding, Y., Griggs, D. J., Noguer, M., van der Linden, P. J., Dai, X., Maskell, K., and Johnson, C. A., Cambridge University Press, Cambridge, UK and New York, NY, USA, 349–416.
4 ESCI 407/507: Forest Ecology Spring (2016), Last updated: 5/17/2016 Lab #7: Carbon Cycling.
5 Dixon, R.K., Brown, S., Houghton, R.A., Solomon, A.M., Trexler, M.C. & Wisniewski, A. (1994) Carbon pools and flux of global forest ecosystems Science 263,5144: 185-90.
6 Anon. (2011) State of the World’s Forests, FAO, Rome.
7. Falkowski, P.; Scholes, R. J.; Boyle, E.; Canadell, J.; Canfield, D.; Elser, J.; Gruber, N.; Hibbard, K.; Högberg, P.; Linder, S.; MacKenzie, F. T.; Moore b, 3.; Pedersen, T.; Rosenthal, Y.; Seitzinger, S.; Smetacek, V.; Steffen, W. (2000). "The Global Carbon Cycle: A Test of Our Knowledge of Earth as a System".Science 290 (5490): 291296. Bibcode: 2000Sci...290..291F .doi: 10.1126/science.290.5490.291. PMID 11030643.
8. Pan,Y., Birdsey,R.A., Fang,J.,Houghton,R. Kauppi, P.E., et al. (2011) A Large and Persistent Carbon Sink in the World’s Forests, Sciencexpress www.sciencexpress.org /14 July 2011, page 1, 10.1126,Science.1201609.
9. Huang,J. and McElroy,M.B. (2012). The Contemporary and Historical Budget of Atmospheric CO2 (PDF). Canadian Journal of Physics 90 (8): 707-716. Bibcode: 2012CaJPh..90..707H. doi:10.1139/p2012-033
10. Betts, R. A (2000).: Offset of the potential carbon sink from boreal forestation by decreases in surface albedo, Nature, 408, 187–190,.
11. Kirschbaum, M. U. F., Whitehead, D., Dean, S. M., Beets, P. N., Shepherd, J. D., and Ausseil, A.-G. E.(2011) Implications of albedo changes following afforestation on the benefits of forests as carbon sinks. Biogeosciences, 8, 3687-3696, doi:10.5194/bg-8-3687-2011. www.biogeosciences.net/8/3687/2011/ doi:10.5194/bg-8-3687-2011 © Author(s) 2.
12. www.geocraft.com/WVFossils/greenhouse_data.html (2007) EMAIL: mhieb@geocraft.com Mar 2007 – Just how much of the "Greenhouse Effect" is caused by human activity?
13. United States Environmental Protection Agency.(2017) Climate Change Indicators: Atmospheric Concentrations of Greenhouse Gases. Climate Change Indicators Retrieved 2017-01-20.
14. Forster,P.,Ramaswamy,V., Artaxo, P., Berntsen,T., Betts,R. Fahey,D.W. et al. (2007) Climate Change 2007: The Physical Science Basis. pp.131-217 in: Changes in atmospheric constituents and in radiative forcing. Contribution of Working Group I to the Fourth Assessment Report of the Intergovernmental Panel on Climate Change, Eds. Solomon S., D. Qin, M. R. Manning, Z. Chen, et al.Cambridge University Press, UK.
15. Joos, F., Bruno, M., Fink, R., Siegenthaler, U., Stocker, T. F., Le Quere, C., and Sarmiento, J. L. (1996): An efficient and accurate representation of complex oceanic and biospheric models of anthropogenic carbon uptake, Tellus, 48B, 397–417.
16. Tian He, Quanqin Shao, Wei Cao, Lin Huang and Lulu Liu (2015). Satellite-Observed Energy Budget Change of Deforestation in Northeastern China and its Climate Implications. Remote Sensing 7, 11586-11601; doi:10.3390/rs70911586.
17. Leathwick, J. R., Wilson, G., and Stephens, R. T. T. (2002) Climate Surfaces for New Zealand, Landcare Research Contract Report: LC9798/126, pp.1-22, available at: http://www.landcareresearch.co.nz/databases/lenz/ downloads/Climate Surfaces for New Zealand revised.pdf, last accessed: 7 March 2011.
18. Pongratz,J. Reick,C.H., Raddatz,T. Caldeira, K. and Claussen, M. (2011), Past land use decisions have increased mitigation potential of reforestation. Geophysical Research Letters, 38, Issue 15, August, DOI: 10.1029/2011GL047848.
19. Jones,A. and Hawkes,D. (2015) Radiative Forcing, Albedo in Land-Use Scenarios. ESD News and Events on May 11, Climate Sciences Department Research Highlight Terrestrial Ecosystem Science Program, University of California, Berkeley. http://link.springer.com/article/10.1007%2Fs10584-015-1411-5#page-1
20. Jones, A.D., Calvin,K.V., Collins,W.D. and Edmonds ,J.(2015), Accounting for radiative forcing from albedo change in future global land-use scenarios. Climatic Change, DOI 10.1007/s10584-015-1411-519. Rahmstorf, Stefan (2008). "Anthropogenic Climate Change: Revisiting the Facts". In Zedillo, E. Global Warming: Looking Beyond Kyoto (PDF). Brookings Institution Press. pp. 34–53.
21. Falloon, P. D., Dankers, R., Betts, R. A., Jones, C. D., Booth, B. B. B., and Lambert, F. H.(2012) Role of vegetation change in future climate under the A1B scenario and a climate stabilisation scenario, using the HadCM3C Earth system model, Biogeosciences, 9, 4739-4756, doi:10.5194/bg-9-4739-2012.
22. Breuer, L., Eckhardt, K., and Frede, H. G. (2003) Plant parameter values for models in temperate climates, Ecol. Model., 169, 237–293.
23. Hollinger, D. Y., Ollinger, S. V., Richardson, A. D., Meyers, T. P., Dail, D. B., Martin, M. E.,et al.. (2010) Albedo estimates for land surface models and sup- port for a new paradigm based on foliage nitrogen concentration, Global Change Biol., 16, 696–710.
Figures
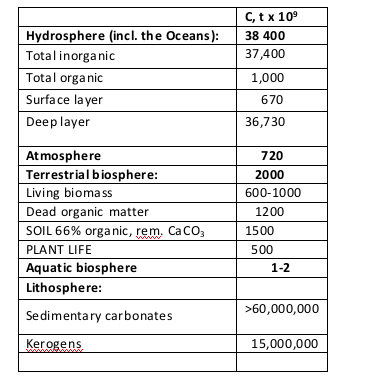
Table 1. Estimated Carbon pools in the major reservoirs (sinks) on earth.(7)
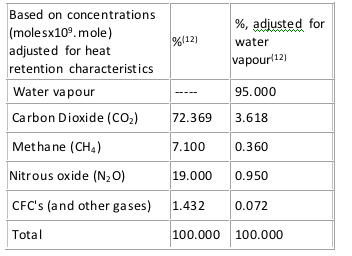
Table 2. Role of Atmospheric Greenhouse Gases as % of Relative Contribution to the "Greenhouse Effect" (12, 13)
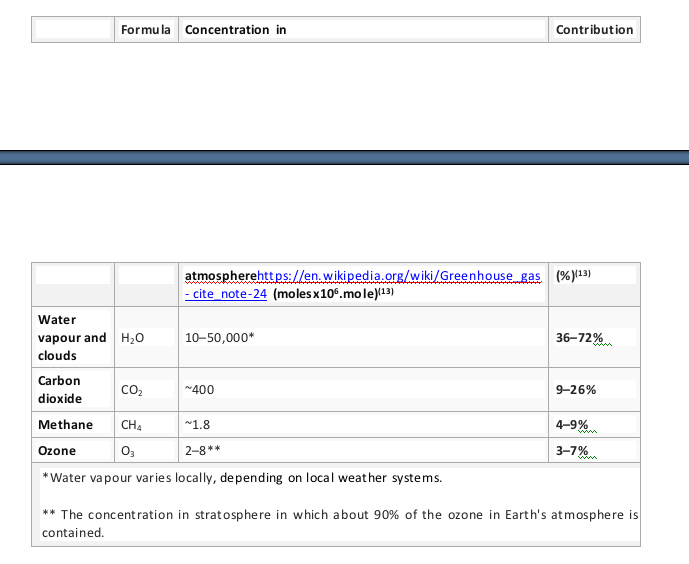
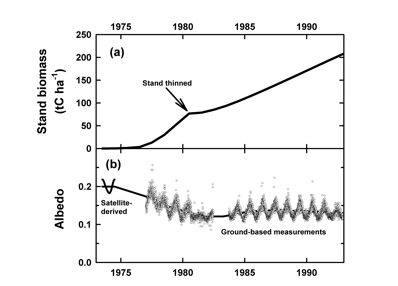
Fig. 1. Changes in forest stand biomass carbon (a) and albedo (b) measured at Puruki, (NZ) and satellite-derived albedo estimates for pastures. Pasture albedo is plotted for 1973 (when the stand of P. radiata was established), but actual measurements correspond to the mean of observations from 2000 to 2008. Also shown are 365-day running means of forest albedo, and a linear interpolation from the pasture data at the end of 1973 to the beginning of the trend line for the forest data. Biomass data include estimated carbon stocks in litter and roots(11).
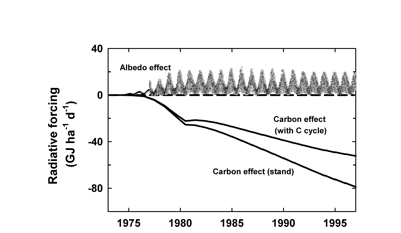
Fig. 2. Calculated radiative forcing due to changes in albedo and carbon storage. Albedo-based forcing is calculated daily from observed albedo and incident solar radiation. Carbon dioxide is distributed globally so that there are no daily variations. Carbon-based calculations were done with considering stand-level carbon storage only (stand), and after calculating carbon cycle feedback effects (with C cycle). The stand was thinned in 1980 (11) .
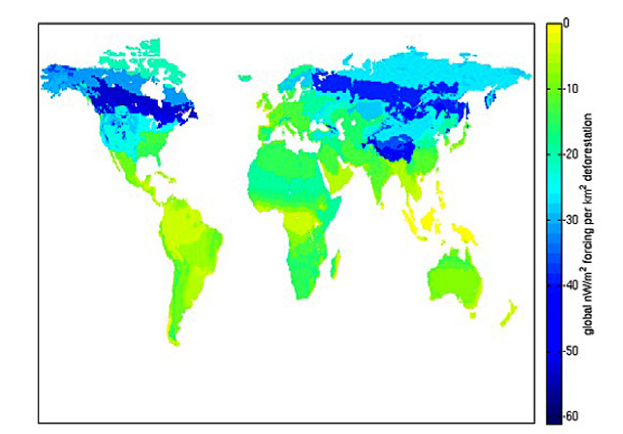
Fig. 3. Radiative Forcing (RF), Albedo in Land-Use Scenarios- the decrease in RF with the
potential replacement of forest by crops or grassland.
Source: Andy Jones and Dan Hawkes(18)