Soil Phosphorus – from feast to famine
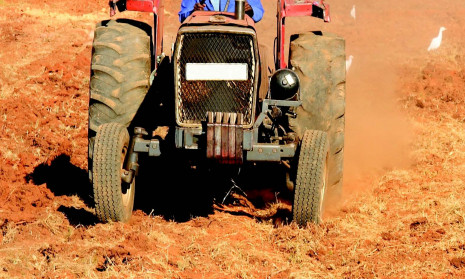
Summary
In the West and in rapidly developing countries, for instance China, the overuse of phosphorus (P) in fertilisers and manures can cause serious eutrophication. In Sub-Saharan Africa the opposite problem – an acute shortage of P- is a more common occurrence. Here we highlight both problems and suggest some possible solutions.
Almost all rivers, streams, ponds or lakes in the UK shows signs of eutrophication. Eutrophication firstly reveals itself as excessive weed and algal growth, such as a thick layer of duckweed floating on the surface of the water, blocking out nearly all light, especially in slow moving canals and sheltered ponds . In extreme cases, there might be blooms of Cyanobacter, or blue-green algae, that can release powerful toxins which are poisonous to both animals and humans. Sometimes fish death might also result. Blooms of blue-green algae closed some large midland reservoirs in the UK for water sports a few summers ago. Agriculture has been the dominant source of polluting nutrients entering the water and is the most likely cause of this proliferation of water weeds and other problems, although it has not been possible to quantify the amounts (Marien, 1997). Of the main nutrients, nitrogen (N), phosphorus (P) and potassium (K), usually referred to as ‘NPK’, excess P is nearly always the main cause and phosphorus concentrations as small 10 to 25 parts per billion (ppb, 10-9) in water can trigger eutrophication. It is usually the ‘limiting nutrient’, meaning that even if N (usually as nitrate) enters water, it will be used only by aquatic plants for growth if sufficient P is already available. As soon as the P has been taken up, further N will not cause any more growth until a further pulse of P, either from recycling within the ecosystem, or as a fresh input from any source, enters the water body.
Soils from natural ecosystems, such as heaths and forests normally contain only very small concentrations of P. It is tightly recycled under these conditions and little leaves the soil–plant system to enter ground water. However, agriculture releases large amounts of P in inorganic and organic forms as runoff from unused fertiliser, animal manures and other organic wastes. Sewage treatment plants represent another source, as treatment units invariably release some P in the effluent passed directly into rivers, regardless of the care applied to control P loss.
Most soils have a very high ability to fix P, which then accumulates in the surface soil. Nevertheless, it has long been known that P which is adsorbed on small soil particles can move along the soil particles by surface flow or as P dissolved in rainwater and as undissolved particulates. It may also move via the wind as P adsorbed on dust particles. Indeed, thousands of years ago the Chinese ploughed across hill slopes rather that down them to avoid soil loss (and, although they did not know it, to avoid P loss as well). Nitrate can also leach down the soil profile into ground water. However, unlike nitrate, P has long been considered to be too tightly fixed in soil to leach, except in a few special circumstances (extremely sandy or highly organic soils) or where very large amounts of organic manures have been applied recently.
New results from a 160-year-old experiment – Broadbalk
Over the last decade or so we have had to change our views on P leaching from soils as new results from the famous Broadbalk experiment at Rothamsted have emerged (Heckrath et al 1995). This experiment, established in 1840, is now the longest running field experiment in the world. It was started by Sir John Bennett Lawes and Sir John Gilbert to demonstrate to farmers that agricultural crops did not need, as was thought then, farmyard- or other manures to grow. Instead, if the correct amounts and combinations of inorganic N, P and K were given, exactly the same yields of wheat or other crops could be produced from either organic or inorganic sources. This finding was a true breakthrough and literally transformed agriculture overnight. For the first time, farmers were freed from the problem of obtaining and handling bulky and scarce animal manures and could use simple inorganic salts instead. Indeed, local farmers were so grateful to Lawes and Gilbert that they commissioned a new laboratory for them to carry on their research.
The experiment, although modified frequently to keep up with modern agricultural practices, would still be recognisable to Lawes and Gilbert. Although receiving inorganic fertilisers for 160 years and being ploughed to 23 cm depth annually, some plots, given optimum rates of fertilizer and manures, have now given annual yields over 11 tonnes of wheat grain per hectare. There is no sign of biological, chemical or physical damage to the silty clay-loam (Batcombe series, plateau drift and clay with flints ) soils of Broadbalk and wheat has been grown virtually continuously since 1840, a finding which confounds the ‘organic farming’ lobby who proclaim the needs to rotate crops and provide animal manures as the source of fertilizer. Each treatment consists of a single plot (the need for replication was not understood when the experiment was initiated a hundred sixty years ago) of 0.2 ha, running from the top to the bottom of the field. The fertilizer and manure treatments are much as before, although maximum N rates are now larger and modern pesticides and wheat varieties are used. Even now the nil plot, which has received no fertilizer of any sort since the start, still produces up to about the world average of 2.7 t grain ha-1.
To date, more than 330 scientific publications have stemmed from scientific research done on the soils and crops of this remarkable UK field (Fig. 2). The statistical techniques of analysis of variance, Student ‘t’ test and randomised block design, which are used world wide in modern field experiments, and in many other ways, all stem from the pioneering work of the Rothamsted statisticians, the late Ronald A. Fisher and Frank Yates.
They perceived the needs for sample replication at the field and laboratory scale and for determining if apparent differences between treatments were due to chance or had real meaning (i.e. were they statistically different).
Anyone who works at Rothamsted simply has to marvel at the insight of Lawes and Gilbert. Lawes was unable to finish his degree at Oxford owing to the death of his father but that did not stop him becoming one of the intellectual giants of his age (Dyke, 1983). Many of Lawes’ and Gilbert’s findings are as valid now as ever they were, even though in the 1840s it took them, or their assistants, a whole day to measure the potassium concentration of a single soil sample.
In 1995, we began working on soil P problems again, after a break of many years. Previously, Rothamsted had focussed on the value of P as a nutrient for agricultural crops. Its possible role as a pollutant of surface and ground waters was not then recognised. However, as evidence of decreasing water quality in the UK increased, it was realised that P may be involved. Remarkably, when Lawes and Gilbert established the field plots of Broadbalk they inserted a field drain at 65 cm below the soil surface and along the centre of each plot (not replaced until 1993) so that they could collect their drainage waters when the soils became wet and reached field capacity during the winter. The soils of Broadbalk gave a unique opportunity to study possible P losses in drainage water from soil to ditch and ground water. In this field each plot receives different rates of applied P fertilizer and different amounts of P are removed by the various wheat crops. Analyses of water from the drain under each plot, has shown each to contain a different concentration of plant-available P as Olsen P (about 5 – 110 mg 0.5 M NaHCO3 extractable P kg-1 soil) in the plough layer (Heckrath et al., 1995). No-one had previously systematically studied possible P losses in water from the Broadbalk drains. First, as explained above, research at Rothamsted had focussed on P as a fertilizer. Probably more importantly, the scientific literature made it clear that P did not leach, other than in exceptional circumstances, so there would seem little point in measurement of drainage losses of P.
The total P concentrations in the dra- inage water from under some of the ‘high P’ plots were over 3 mg PL-1; concentrations well over a hundred times more than is required to cause eutrophication. This was in stark contrast to all of the previous published data (Heckrath et al., 1995).
The P in the drainage waters was measured at different stages in the rainfall cycle, depending upon time of rainfall, working hours etc. The concentrations of P in drainage waters therefore varied quite widely even under the same plot, depending upon when the water was collected. However, P started to move from soil to drainage water only when a specific soil P concentration was reached – termed the Change-Point- and this was quite independent of the drainage event. For Broadbalk the Change–Point was about 60 mg P kg-1 soil. The question was, did different soils have an identical Change-Point, and was this the same as for Broadbalk? Whether the Change-Point was the same for other soils, or different from that of Broadbalk soils, it would indicate the soil P concentration above which there would be significant risk of P leaching losses.
Unfortunately, to test this we would have needed to measure P in drainage waters from soils of different types (ranging from sandy to heavy clay) with soil P gradients from high to low concentrations – and there are very few, if any, sites with these features in Europe and certainly not in the UK. Furthermore, to collect drainage water throughout the UK in this way would have been time consuming and difficult, as we would have been totally dependent on rainfall events in the ‘leaching season’- typically from October to April. Even then it is likely the drains would have worked only for a few hours at any time, depending upon rainfall intensity. Also the waters need to be chemically analysed within hours of collection to avoid chemical changes.
In order to extract P from soil in a way that simulates the natural process, with an action approximating to that of soil solution or rainfall, and to avoid the collection of drainage water a dilute (0.01 M) calcium chloride solution has long been used as an alternative, ‘weaker’, soil extractant than Olsen’s reagent. Data from Johnston and Poulton(1993) for inorganic P soluble in 0.01M CaCl2 (CaCl2-P) and Olsen P for Rothamsted soils (including Broadbalk) were replotted and gave a relationship closely akin to that of the Broadbalk drainage water-P data (Brookes et al 1997). This indicated that laboratory extractions of Olsen P and CaCl2-P could be used to indicate if the Change-Point differed between soils (Figure 3, below).
Further work, on a range of soils from the UK, indicated considerable variations in Change-Points in different soils, measured as described above, i.e. under laboratory conditions, as these Change-Points ranged from about 29 to 110 mg Olsen P kg-1 soil (Hesketh and Brookes, 2000). Later, other work (Fortune et al. 2005) estimated that maximum total P losses from agricultural soils caused by leaching down the soil profile were estimated to be around 2 to 5 kg P ha-1. While of no economic importance to the farmer, this is a significant input to the environment which needs to be curtailed to decrease eutrophication. Fortunately, most arable crops reach a yield -1 maximum at below 25 mg P kg so if soil P concentrations can be maintained at around this level, P leaching losses from soil to water can be minimised, without any decrease in yield. However, many vegetables require much larger soil P concentrations for maximum yield and animal manures are mainly disposed of to agricultural land. The net result is that average soil P concentrations of UK agriculture soils in 1993 were increasing by about 15 kg P ha-1 y-1, posing a considerable risk to water quality in UK. Since then, soil P concentrations have decreased slightly, due to decreased fertilizer use (Withers et al., 2001).
Phosphorus over-use in China
Over the last decade, China has had the fastest increase in economic activity of any country of the world, with growth rates approaching 10 % per year. Agriculture has developed rapidly to match food demand, particularly around centres of rapid population growth, such as Beijing and other major cities. With a total human population currently estimated at 15.2 million, Beijing alone has brought its own environmental problems.
It is widely recognised by Chinese agronomic advisors and scientists that overuse of fertilisers and manures is an increasing agricultural problem . This is partly due to lack of information available to the farmer but also due to the sheer scale of animal production, especially that of pigs, in the peri- urban areas around Beijing and other major cities. Pig farms in this region are highly intensive, often with 4000 pigs per farm. Not surprisingly therefore these farms face major problems in manure disposal. In many cases it is applied directly to agricultural land for vegetable crops. However, inorganic fertiliser is also frequently applied, with the result that soil P concentrations can reach very high concentrations. For example, in some Chinese horticultural soils Olsen- P has increased to several hundred mg P kg-1 soil (Lin, 2006). Until recently, pig manure was invariably stored dry or in liquid forms in lagoons until it could be disposed of (Fig. 4). The risk of nutrients entering rivers and lakes from this source is self-evident and most water sources in China show much evidence of eutrophication from over-use of manures and fertilisers. One good step forward is that some of the larger pig farms are now pelleting pig manure after machine drying and it is being bagged and exported to other areas as an organic fertiliser (Liu et al., 2007)
Nevertheless the problem of over use of manures and fertilisers remains and, overall, soil P is accumulating in many agricultural regions. The inorganic chemical P fertilizer imported into agricultural soils of China has increased greatly during the last decades. About 1.2 million tonnes P fertilizer was applied in 1980, increasing by 7 % annually from 1980 to 1985, then by 12 % from 1986 to 1995, reaching 6 million tonnes by 2003 (Lin, 2003). Currently, China is the largest consumer of P fertilizer in the world, using 30% of the total production in 2002 (Prud'homme and Heffer, 2004). This problem of P accumulation in Chinese agricultural soils and its environmental implications is now recognised in China. Accordingly, several research projects have operated over the last few years by the Chinese Agricultural University, Beijing, led by Professor Qimei Lin, the Institute of Sub-Tropical Agriculture, led by Professor Jinshui Wu, The North-West University of Agriculture and Forestry, led by Professor Jialong Lu and Rothamsted Research, led by Professor Phil Brookes, UK. Chinese funding has come from Academica Sinica and the Chinese Academy of Sciences and UK funding from the Biotechnological and Biological Sciences Research Council, the British Council and the Royal Society.
The Chinese and the UK face broadly the same problems with P. However, because of high population pressure in large industrial cities and the massive overuse of manures and fertilizers in some areas, the Chinese problems are especially acute. Research has focused on determining rates of accumulation of P in Chinese agricultural soils, soil P concentrations at which P leaching might begin (the Change- Point), the soil P chemistry involved and a better understanding of mechanisms of P loss from soils to water.
There have been regular recent exchanges between UK and Chinese scientists and, recently, several Chinese scientists have worked in Phil Brookes’ group at Rothamsted for periods of up to a year. Dr. Xiaorong Zhao from Professor Lin’s research group at the Chinese Agricultural University (CAU), Beijing, recently worked with Phil Brookes at Rothamsted and Dr. Kathy Snars from 2006-7 in a joint BBSRC- CAU project to better understand the role of the soil microbial biomass in P leaching losses in UK upland, winter- waterlogged and Chinese paddy soils. This work is also being done jointly with the Institute of Grassland and Environmental Research, Devon, UK. In October, 2007 Miss Yuping Yu from Zhejiang University and Miss Li Chunyue from Northwest Agricultural and Forestry University, Shaanxi joined the group for 1 year. In January, 2008 Dr. Shengli Guo, also from Northwest University, joined us too as a Rothamsted International Fellow.
The soil microbial biomass can be described as a large pool of potentially available P (c.a. 100 kg P ha-1 in UK grassland, less in arable soils) (Brookes et al., 1984). This P is potentially plant-available but might also contribute to P leaching losses during the process of biomass turnover (Kouno et al., 2002). Air-drying and rewetting releases considerable amounts of P from the biomass, which may possibly contribute to P losses from soils to water (Turner and Haygarth, 2001). Waterlogging of soils (e.g. in Chinese paddy soils), also releases a significant quantity of P from the biomass (Xiaorong Zhao, unpublished data).
The aim of this joint research is to provide scientific advice to farmers in intensive and small scale agricultural systems on environmentally safe upper limits of P which should be permitted to accumulate in Chinese soils. At present it is very difficult to persuade these farmers to cut back on P fertilisation. In pig-growing areas it is normal practice for farmers growing vegetables to supply soils with liberal amounts of pig manure and then to apply additional inorganic fertiliser, apparently as a precautionary measure. Our work in the UK and China has shown that soils can only retain so much P before the Change- Point is reached and then, in addition to surface losses from erosion and runoff, P will start to move down the soil profile into ground water. In some regions, especially peri-urban areas, where manure production is excessive, it seems likely that this process is happening already. Hopefully our research will help to mitigate the problem but the current situation is analogous to China sitting on a ‘Phosphorus Time Bomb’!
Soil phosphorus – the bottleneck of world hunger
Much of the West and developing countries such as China are experiencing environmental problems caused by excessive use of P fertiliser. However, in Sub-Saharan Africa many soils, particularly those of small scale farmers, are nutrient depleted, owing to nutrient removal in crop produce exceeding nutrient inputs.
Phosphorus is often the limiting nutrient for plant growth and it has been aptly termed ‘the bottleneck of world hunger’ (Rorty, 1946). N, can be fixed by legumes from atmospheric N2. By contrast, to increase soil P availability it is necessary to add P directly, as inorganic fertilisers, composts or manures. These are generally scarce and, in the case of P fertiliser, cost can inhibit its use. In addition, many soils in this region have extremely high P fixation capacities and even if P is applied to the soil it can be ‘locked up’ by complexing with Fe or Al and can be almost completely unavailable to the growing crop. Crops grown in nutrient deficient soils are especially susceptible to attack by parasitic plants (Striga spp.) which can literally destroy a crop.
Collaborative work between the Kenyan Agricultural Research Institute (KARI) in Nairobi, Kenya and Rothamsted Research is investigating the mechanisms involved with a view to devising practical methods to increase soil P availability in this situation. Two approaches were adopted. First, by attempting to saturate the P-fixing sites in the soils through application of a large annual application of P (75 kg P ha-1), that should serve for several seasons; and second, by attempting to keep the fertilizer P in biological forms through the supply of fertilizer P and cattle manure (FYM) in combination. Here, the aim was to promote the cycling of P through the soil microbial biomass and associated metabolite pools, with an expected result of decreasing P fixation and increasing plant availability of this P.
These treatments were investigated at two field sites on smallholder farms in Kenya: one, considered to be a ‘high P fixing’ soil (ferro-orthic acrisols) at Malava (Kakamega District) and one considered to be a ‘low P fixing’ soil (mollic andosols) at Mau Summit (Nakuru District). The following five treatments were applied in 1997 and 1998: nil; 75 kg P ha-1 as super-phosphate (P); 25 kg P ha-1 as superphosphate; FYM at 1.9 t ha-1 dry matter; 1.9 t FYM plus 25 kg P ha-1. All treatments also received 100 kg inorganic N ha-1. Maize was the test crop. There was no significant statistical correlation in either year at either site between soil P and maize yield, measured as NaHCO3- extractable P, anion resin-extractable P or NaOH-extractable P. However, the results for the different soil P extraction methods (fractions) were closely correlated.. Yields at the high P rate (75 kg ha-1) were often little better than those of the control. There was, however, a significant positive relationship (P<0.05) between soil microbial biomass P and crop yield, at both sites and in both years. The treatment giving the highest grain yield and the largest soil biomass P was always FYM plus P (Ayaga and Brookes, 2006). The results indicated that the combined use of organic and inorganic fertilizers in these low input systems can promote increased biological cycling, enhanced availability and consequently improved plant uptake of soil and fertiliser P, to the advantage of the small scale farmer (Fig. 8). The results also indicated that biomass P measurements might provide a better indicator of soil P availability in these soils than some more conventional chemical procedures.
The aim now is to obtain further funding to test these approaches in conjunction with smallholders to see if they are also useful under normal farming conditions in Kenya and other Sub-Saharan African Countries
References
I Ayaga, G. & Brookes, P.C. (2006). Enhanced biological cycling of phos- phorus increases its availability to crops in low-input sub-Saharan farm- ing systems. Soil Biology & Biochemistry.
I Soil Biology and Biochemistry. 38, 81-90.
I Brookes, P.C., Powlson, D.S. & Jenkinson, D.S. (1984).Phosphorus in the soil microbial biomass.
I Soil Biology and Biochemistry 16, 169-175.
I Brookes, P. C., Heckrath, G., De Smet De J., Hofman. G., Vanderdeelen, J. (1997) Losses of phosphorus in drainage water. In: Phosphorus Loss from Soil to Water. (Eds. H. Tunney, O. T. Carton, P.C. Brookes, A. E. Johnston). CAB International. 253-271.
I Dyke, G. (1983)
I John Lawes of Rothamsted: pioneer
of Science, Farming and Industry. I Hoos Press, Harpenden, UK.
I Fortune S., Lu J., Addiscott, T. M., Brookes P. C. (2005)
I Assesment of phosphorus leaching losses from arable land.
I Plant and Soil. 269, 98-108.
I Hesketh, N., Brookes, P.C. (2000)
I Development of an indicator for risk of phosphorus leaching.
I Journal of Environmental Quality. 29, 105-110.
I Heckrath, G., Brookes, P.C. Poulton, P.R., Goulding, K.W.T. (1995) Phosphorus leaching from soils con- taining different P concentration in the Broadbalk experiment.
I Journal of Environmental Quality 24, 904-910.
I Johnston A. E., Poulton P. R. (1993)
I The role of phosphorus in crop pro- duction and soil fertility: 150 years of field experiments at Rothamsted, United Kingdom. In: Phosphate Fertilizers and the Environment. (Ed. J. J. Schultz) International Fertilizer Development Center, Muscle Shoals, Alabama, pp. 45-63.
I Kouno, K., Wu, J. ,Brookes, P.C. (2002)
I Turnover of biomass C and P in soils following incorporation of glu-
cose or ryegrass.
I Soil Biology and Biochemistry 24, 617-622.
I Lin, Q. (2003).
I The situation and prospect of Chinese phosphorus fertilizer industry (in Chinese).
I China Petroleum and Chemical Industries, 1, 38-39
I Zhao X., Li G., Lin Q.?2008?Interference of soil extractable phosphorus in measuring soil microbial biomass phosphorus. Communications in Soil Science and Plant Analysis, 39: 1367–1374.Liu X., Luo Q., Feng Z.Zou S., Liu G. , Liu Y. (2007)
I Commercial organic fertilizer in China (in Chinese). Acta Agricultural Jiangxi 19, 49-52.
I Marien F. (1997) European perspec- tive on phosphorus and agriculture. In: Phosphorus Loss from Soil to Water. (Eds. H. Tunney, O. T. Carton, P. C. Brookes) A. E. Johnston CABI
I Prud'homme, M., and Heffer P. (2004)
I World Agricultural Situation and Fertilizer Demand, Global Fertilizer Supply and Trade 2004/2005. http://www.fertilizer.org [should we establish a precedent here and request date of access, given that web sites are frequently updated?]
I Rorty J. (1946)
I Phosphorus – the bottleneck of world hunger. Harpers Magazine. 1158, 472-480.
I Turner B., Haygarth P. H. (2001)
I Phosphorus solubilization in rewet-
ted soils.
I Nature, 41, 258.
I Withers P. J. A., Edwards A. C., Foy, R. H. (2001).
I Phosphorus cycling in UK agricul- ture and implications for phosphorus loss from soil. Soil Use and Management 17, 139-149.
Download pdfFigures
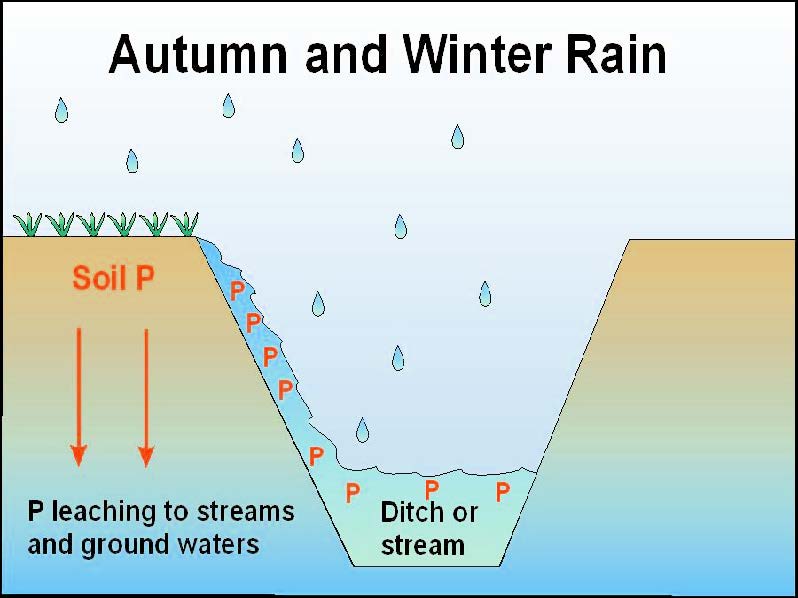
Figure 1 Movement of P from soil to water.
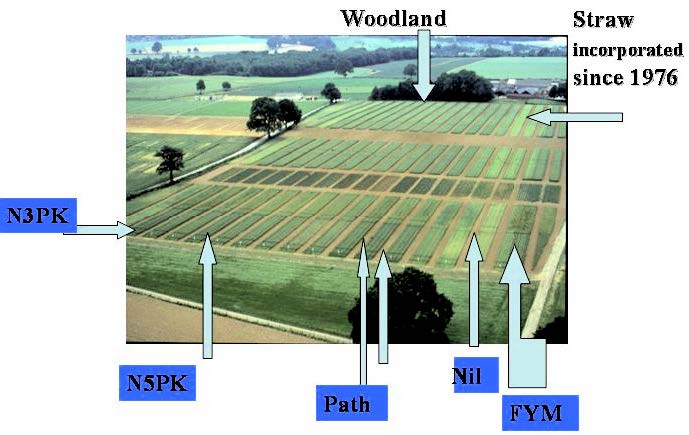
Figure 2 The Broadbalk Wheat Experiment at Rothamsted, showing some of the treatments.
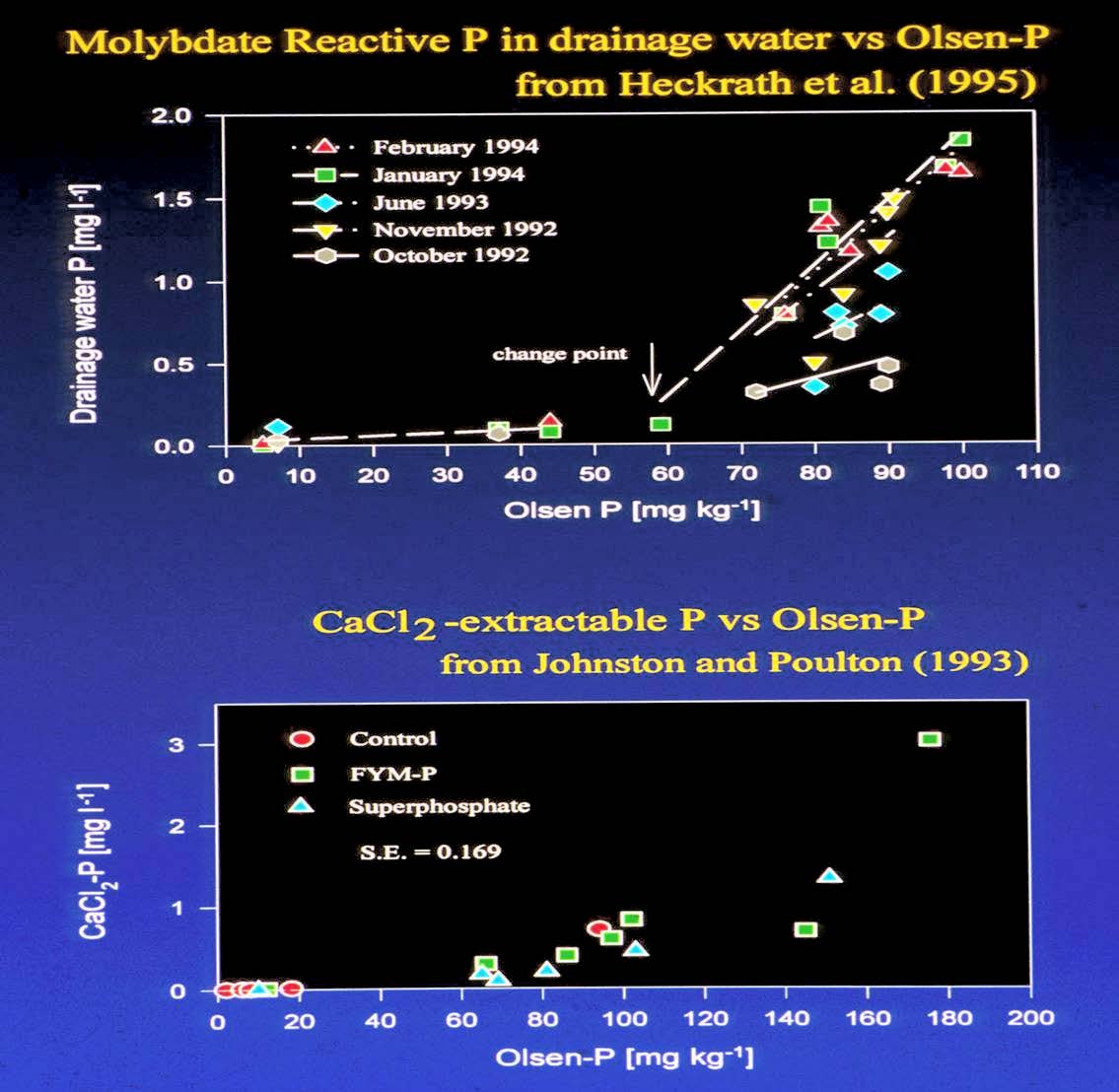
Figure 3 (a) P leaching from Broadbalk during five rainfall events; (b) Relationship between P extracted by CaCl2 and Olsen’s reagent ( Molybdate-extractable P can be considered to be soluble inorganic P).
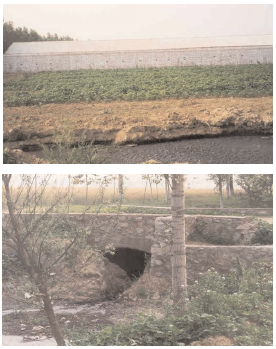
Figure 4 (a) Pig manure slurry and vegetable growing on a Chinese peri-urban pig-farm, Beijing; (b) Stream adjacent to pig farm.
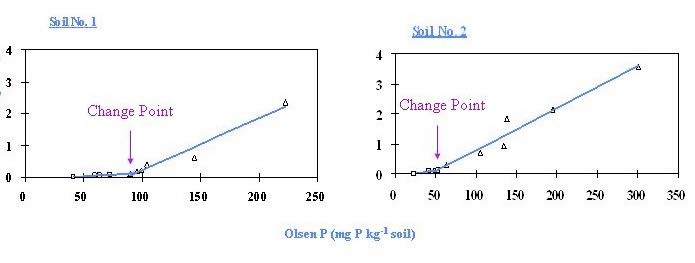
Figure 5 (Modified from Zhao et al. 2007). Change-Point in two Chinese soils. Soil 1 - Red soil: 1.95 % organic carbon, pH 5.5, 52 mg Olsen P kg-1 soil.
Soil 2 – Yellow soil: 2.23 % organic carbon; pH 7.0, 41 mg Olsen P kg-1 soil.
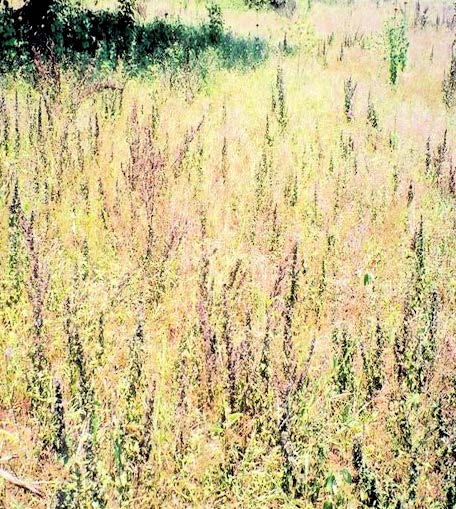
Figure 6 Striga sp.destroying a maize crop in an African farmer’s field.
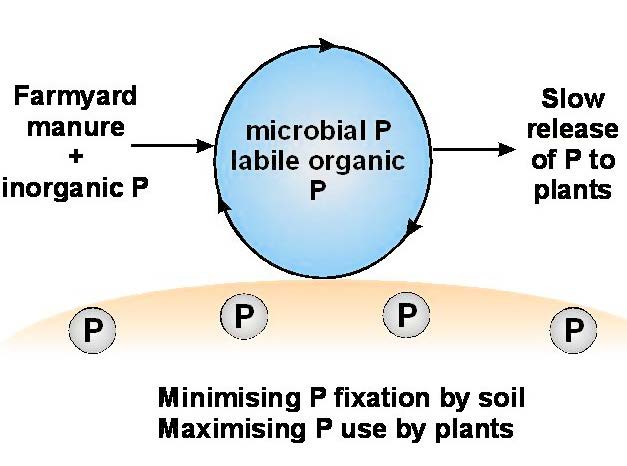
Figure 7 The microbial biomass as a protected reservoir of labile P.
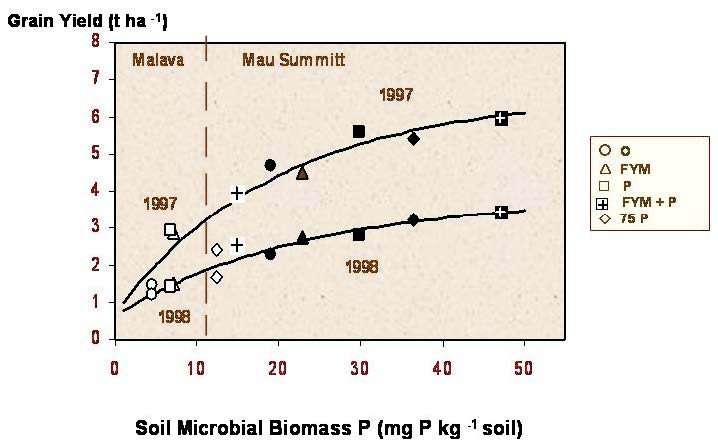
Figure 8 Relationship between microbial biomass P and grain yield attwo Kenyan sites (From Ayaga and Brookes , 2006). White symbols – Malava; Black symbols – Mau Summitt. For treatments see box outside Figure.