Pesticide toxicity and public chemophobia: how toxic are modern-day pesticides?
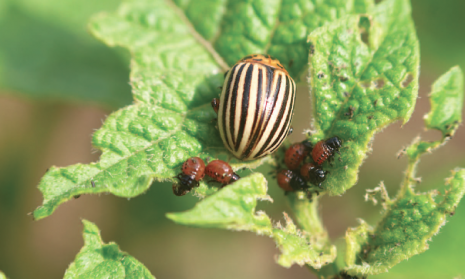
Summary
Global food security is a major issue for the future of humanity. Food demand is predicted to grow strongly for at least the next 40 years and food supply is already struggling to keep up.
Protecting crops from weeds, fungal diseases and herbivorous pests is a critical part of global agriculture and synthetic pesticides are the most effective tools farmers currently have to achieve this.
Nevertheless the public perception of pesticides is strongly negative. In particular pesticides are perceived to be highly toxic and it is widely believed that there are significant negative health implications for people eating food containing synthetic pesticide residues.
This paper shows that modern day pesticides are not particularly toxic relative to other commonly encountered chemicals, both synthetic and natural.
The levels of pesticide residue found in food are many orders of magnitude too low to have any health implications for consumers.
In fact the use of pesticides contributes significantly to food safety due to the reduction of mycotoxin contamination. Consuming food was much more dangerous in the days before pesticides.
Key words: Pesticide toxicity; public perceptions; food security
Glossary
Rat acute oral LD50: the ingested dose of chemical required to kill half the members of a tested population of rats after a specified time.
Acute reference dose: the maximum dose of a chemical taken over a short time period (e.g. a single dose, or over a single day) which appears to be without appreciable risk to the consumer.
Maximum Residue Level (MRL): the maximum concentration of a pesticide that is legally permitted in or on a food commodity.
Acceptable Daily Intake (ADI): the maximum daily dosage of a chemical which, during an entire lifetime of exposure, appears to be without appreciable risk to the consumer.
No Observed Adverse Effect Level (NOAEL): the highest dose of a chemical that resulted in no observable adverse effects on test animals over the complete duration of a controlled study.
Introduction
Humanity is facing a number of profound and unprecedented challenges.
How are we going to satisfy our seemingly insatiable appetite for energy? How are we going to supply enough fresh water to the places that need it? How can we prevent any further catastrophic collapse in global biodiversity? What impact will climate change have and how can we adapt and mitigate against the worst of its effects?
These challenges are all intimately interlinked and they are all linked to perhaps the most immediate and profound challenge we face: how can we produce enough food to satisfy the nutritional needs of the growing world population over the next 50 years?
Food demand is growing strongly. The world population is rising fast, from 6.8 billion people today to an estimated nine billion people by 2050 (see Fig. 1, plotted from data obtained from the FAO, 2011).
Also increasing levels of personal wealth are driving demand for more sophisticated diets, particularly meat and dairy products, which are relatively inefficient in energy terms to produce.
It is estimated that primary global food demand will increase by between 50-100% between now and 2050 (Royal Society, 2009; Godfrey et al., 2010). Others have calculated that more food will need to be produced in the next 50 years than has been produced in the previous 10 000 years combined in order to satisfy demand (One World, 2011).
There is no realistic possibility of simply increasing global farmed area to keep up with food demand.
Indeed we may be fortunate even to retain the area of farmland currently available in the long term.
Almost the entire future rise in global population is predicted to be in urban areas (Fig. 1) and hence cities will have to expand to cope, potentially eating up surrounding farmland.
The fertility of some areas of farmland is being degraded by poor farming practices leading to erosion, salination or compaction of the soil, or chronic depletion of nutrients (Tilman et al. 2002; Foresight 2011).
In the future there may be an increase in the arable area used for non-food crops such as biofuels or the “biorefining” of other feedstocks (Foresight, 2011).
It is also generally recognised that the large scale conversion of wild lands to farmland would have further catastrophic effects on biodiversity, and greenhouse gas emissions associated with land-use change.
* Pests that attack arable crops in the field are becoming more difficult to control. Resistance is developing to many pesticides, reducing their effectiveness or in some cases making them completely obsolete (Denholm et al. 2002; Merotto Jr. et al. 2009).
This is simply a consequence of Darwinian evolution under high selection pressure, exactly analogous to the evolution of antibiotic resistance in bacteria. Occasionally new pest species emerge as significant threats to agriculture.
Two recent examples of new problematic fungal diseases are soybean rust in he Americas (Pivonia and Yang 2006) and a strain of wheat stem rust (Ug-99) in Africa and the Middle East (Zhang et al. 2010).
Superimposed on these biological challenges is the effect of increasingly stringent pesticide legislation. The imposition of tough, arbitrary, hazard-based standards for pesticide safety in Europe is having the effect of removing safe and effective pest control strategies from European farmers, compromising their ability to compete in the global food production market.
A recent report on the impact of these proposals on UK agriculture (ADAS 2008) estimated that wheat production would decrease by 26-62% (depending on the exact scenario implemented), potato production would decrease by 22-53% and brassica production would decrease by 25-77%.
Also the potentially dramatic reduction in the number and diversity of pesticides available will make it much more difficult for farmers to manage the evolution of pesticide resistance which generally relies upon rotating through a range of diverse pest control strategies.
* A number of farm input streams are coming under increasing pressure. Synthetic nitrogen fertilisers take significant amounts of energy to produce and hence their price tends to track the oil price which is rising strongly (Foresight 2011).
Economically extractable supplies of mineral phosphates are declining and are largely concentrated in North Africa. The continuity of phosphorus supply is a matter of some controversy, but some estimates suggest that “peak phosphorus” supply could be reached by around 2030 (Cordell et al. 2009).
Water supply is the limiting factor for agricultural yields in many parts of the world. It is estimated that 70% of the world’s fresh water is used in agriculture and yet it seems that water is becoming increasingly scarce in the areas that need it most.
Already 25% of the world’s rivers do not reliably reach the sea since at times every drop of water is being extracted for industrial, agricultural and domestic uses, and we are mining ancient “fossil” groundwater at an unsustainable rate (Ridoutt and Pfister 2010).
* The global credit crisis has hit farming hard. Farmers rely on credit to invest in the inputs required to grow their crops.
Less available credit means that farmers are less able to invest in more expensive but higher performing seed varieties and crop protection strategies resulting in lower achievable yields (Sanchez 2005).
Similarly farmers in some parts of the developing world find it difficult to insure their crops, making them less likely to invest the money required to purchase premium inputs if the resulting crops may be at risk of damage by uncontrollable circumstances, such as extreme weather events (Thomas 2008).
* Lack of infrastructure is limiting agricultural productivity in some parts of the world.
Poor roads, a lack of proper food storage facilities and suitable vehicles (especially with refrigeration facilities) means that many farmers in the developing world cannot gain fair access to global food markets and huge amounts of food are being wasted.
Also a lack of adequate communication technology infrastructure (mobile phones, internet access) can hinder farmers when they are deciding what crops to grow and how best to grow them (Sanchez 2005).
All of these pressures are underpinned by the uncertainties associated with climate change.
Increasing temperatures accelerate plant maturation and inhibit leaf development and grain filling.
This, in combination with a lack of water, can have a devastating effect on crop yields. In the anomalously hot summer in Europe in 2003 yields of wheat fell by over 20% and yields of maize fell by 30%.
Models predict that by the end of the 21st century, the average summer in Europe will be hotter than the summer of 2003 (Battisti and Naylor 2009). As the climate changes, the zones in which crops can be grown will shift.
Some currently fertile regions are likely to become too hot and dry to support any agriculture at all but on the other hand, some regions which are currently too cold to farm may become fertile.
An International Food Policy Research Institute report (Nelson et al. 2009) attempted to quantify the effect of both yield changes and farmed area on food production as a result of climate change. This report concludes that for the two crops most critical for human consumption, global rice production would fall by 10-15% and global wheat production would fall by 20-30%.
The issue of how we can feed the world’s population over the next 50 to 100 years without utterly devastating the global environment in the process is the most significant challenge humanity faces. It is unlikely that a single “silver bullet” technology will be developed that will provide a complete solution to our needs.
Rather, if we are to succeed it will be through the judicious use of all the technological tools in the agricultural tool box, alongside traditional knowledge and techniques.
These tools should be thoughtfully and appropriately applied in order to optimise the efficiency of agriculture in every individual local situation.
All of our tools have a part to play, it is unfortunate therefore that public opinion is so set against one of the most important and useful tools at our disposal.
The benefits of pesticides
There is universal agreement that farmers must take measures to protect their crops from pests.
Weeds compete with crop plants for soil nutrients, water and sunlight. Plant infectious diseases are usually caused by fungi (or similar organisms), but bacterial and viral diseases are also damaging.
Invertebrate pests feed on the crop, directly impacting on yield and quality, but some can also act as vectors which spread disease. There are numerous pest control strategies used by farmers worldwide.
Agronomic practices are important, for instance crop rotation can suppress plant diseases and invertebrate pests, whilst ploughing and hand weeding can be used to manage invasive weeds.
Biological methods can be used to control insects, for example bacteria which produce insecticidal toxins like Bacillus thuringiensis, entomopathogenic fungi that infect insects, and predatory species such as wasps and ladybirds that attack caterpillars and aphids respectively.
Also the breeding of plants to have intrinsic resistance to fungal and invertebrate pests is critically important. This was traditionally achieved by the tortuous process of random crossing and selection with generally no idea how increased resistance was being achieved.
More recently plants have been genetically engineered to resist pests using more rational and targeted strategies.
However, by far the most significant method for controlling pests on arable crops is the use of chemical pesticides (more accurately – crop protection chemicals).
Pesticides are often used in combination with one or more of the other pest control methods described above but the use of pesticides is usually at the heart of a farmer’s pest control strategy.
To understand why, it is necessary to consider the main benefits the use of pesticides can bring:
The key benefit is increased yield. Pesticides remain the dominant and most effective mechanism to control pests, and it has been estimated that the use of crop protection technologies increases global crop yields by 92% (calculated from Oerke et al. 1994).
Without crop protection the world would be able to produce little more than half of the food currently produced. More specifically crop protection increases global yields of wheat and rice by 43% and 172% respectively (calculated from Oerke 2006).
* Pesticide use can also improve the quality of the harvested crop, ensuring that the food we eat is not damaged by insects or blemished by disease whilst in the field or after harvest.
* Pesticide use can improve the safety of our food through the suppression of pests and diseases which can contaminate crops with natural toxins (see below).
* The use of pesticides has a direct beneficial effect on global biodiversity by helping humanity to produce the food it needs without having to convert large areas of pristine wild land to farmland.
A number of studies have examined how best to maximise biodiversity whilst producing a given amount of food from a given amount of land. This raises the question: is it better to farm the whole area “ecologically”, or is it better to farm a proportion of the area “intensively” and allow the remainder to revert to wild land?
The answer is clear: wild land is typically much more biodiverse than any farmland, and the differences in biodiversity between ecological farmland and intensive farmland are generally smaller than the differences in crop yield.
This means that it is usually better to farm the existing land intensively rather than convert wild land to farmland (Green et al. 2005; Hodgson et al. 2010; Benton et al. this issue).
* Use of pesticides provides economic and social benefits as they help to keep food prices relatively low.
It has been estimated that without pesticides food prices in the UK would rise by about 40%, lowering family disposable income with knock-on effects on the quality of family life. It is also likely that rates of consumption of fresh fruit and vegetables would decrease resulting in a detrimental impact on public health (Rickard 2010).
In 1994 it was estimated that the amount of additional food produced owing to crop protection was worth $160 billion (Oerke et al. 1994).
The global crop protection market that year was worth around $28 billion, so on average for every dollar invested on crop protection, the farmer recouped over $5.50 in increased yield and quality, an impressive rate of return.
Despite these benefits the general public has a strong negative perception about the use of pesticides and especially their impact on the health of people who consume food containing pesticide residues.
The latest UK Food Standards Agency Consumer Attitudes Survey (FSA 2008) reveals that when prompted 32% of consumers state that they are concerned about the use of pesticides to grow food.
It is very easy to find scare stories in the media and on the internet of pesticide exposure being “linked” to a wide variety of dreadful diseases and medical conditions such as cancer, neurodegenerative diseases, sterility and diabetes (see Box 1).
The clear implication being that eating food containing pesticide residues will increase the chances of contracting such a disease.
However this is completely at odds with the fact that there are no recent verified reports in the scientific literature of negative impacts on human health owing to the consumption of pesticide residues in food.
The relative toxicity of pesticides
The foundations of modern toxicology were laid over 500 years ago by the Swiss physician Paracelcus who famously said (Wikipedia 2011a):
“All things are poison and nothing is without poison; only the dose permits something not to be poisonous”
In other words any substance can be poisonous if the dose is high enough, and even the deadliest toxins can be completely benign if the dose is small enough.
It would seem that most members of the public have a strong negative perception about what pesticide residues in their food are doing to their health.
Most people have little idea how toxic modern day pesticides actually are relative to other compounds to which they are commonly exposed, nor do the public generally know how much pesticide residue they are actually exposed to in their diets.
An objective examination of these two factors is instructive.
In Fig. 2 (below) the toxicity of the world’s best selling pesticides in 2009 is plotted against the year of first introduction of the pesticide.
The measure of toxicity used is the rat acute oral LD50 (see Glossary), which is the most common measure of toxicity used in scientific literature.
It might be argued that an analysis of chronic toxicity would be more relevant to the discussion of pesticide residues in food, but in fact a comparison of acute toxicity should be a tougher test since pesticides are designed to show high levels of acute toxicity to their target organisms.
The top dotted line across the graph represents the toxicity of the over-the-counter medicine ibuprofen (rat acute oral LD50 of 636 mg/kg, (Science Lab 2011a; Oxford Univ. 2011a)).
Ibuprofen is not generally regarded as a particularly “toxic compound” and yet an examination of the graph shows that it is more toxic than all but 13 of the world’s best selling pesticides.
Moreover, it is more toxic than all but two of the world’s major fungicides (metalaxyl and cyproconazole) and all but one of the world’s major herbicides (paraquat).
A good answer to the question “how toxic are modern day pesticides?” is that they are about as toxic as over-the-counter medicines.
The level of pesticide residue present in a normal adult diet is approximately 0.1 mg/day, roughly equivalent to a single grain of fine sand (Ames and Gold 2000).
That is the total cumulative exposure to all pesticides, not exposure to each individual one. An examination of any packet of ibuprofen will reveal that patients are advised nottoexceedadoseof1200mgof ibuprofen in 24 hours.
To consume an equivalent dose of pesticide residue through eating a normal diet at only 0.1 mg of pesticide residue per day would take 12000 days, which is over 32 years!
Taking ibuprofen over the course of several days to alleviate the symptoms of a common cold, for example, can easily lead to more than the equivalent of a lifetime exposure to pesticide residues in food. And yet if ibuprofen was a pesticide it would be amongst the most toxic pesticides in major use today.
The lower dotted line on the graph indicates the toxicity of caffeine (rat acute oral LD50 of 192 mg/kg, (Fisher Science, 2011; Oxford Univ, 2011b)).
Caffeine is almost as toxic as paraquat (rat acute oral LD50 of 129-157 mg/kg), and only a handful of the world’s most toxic major insecticides are significantly more toxic than caffeine.
And yet dietary exposure to caffeine can easily be many thousands of times greater than our exposure to pesticide residues in our food. A single can of cola contains over 30mg of caffeine so it would take about a year to consume the same amount of pesticide residue eating a normal diet.
A single cup of coffee can contain over 100 mg of caffeine (Wikipedia 2011b). Between 2000 and 2008, 721 people were admitted to the Emergency Department of the Royal Infirmary of Edinburgh suffering from a toxic overdose of caffeine (Waring et al., 2009).
Another remarkable fact is that sodium chloride (common salt) is close to the median level of toxicity of the world’s best selling pesticides.
The rat acute oral toxicity of salt is 3000 mg/kg (Oxford Univ. 2011c; Science Lab 2011b) which means that by this measure it is more toxic than 19 of the 44 pesticides considered in this analysis.
The UK National Health Service estimates that the typical consumption of salt in the UK is around 9.5 g per day, an exposure level approximately 95 000 times greater than typical exposures to pesticide residues in food. The maximum recommended daily dose of salt for adults is 6 g per day (NHS 2011).
The hazards associated with pesticide toxicity
It is very difficult to find verified cases of poisoning through ingestion of pesticide residues in food in the peer reviewed scientific literature (a conclusion also reached by Hall 1992).
There were a number of cases reported in the 1950s and 1960s of poisonings owing to the consumption of pesticide contaminated grain, but it seems that in every case the contamination was either accidental (occurring post-harvest) or through fungicide treated seeds intended for planting ending in the human food chain (WHO 1990).
Whilst the situation has been clear in recent times, it has not always been so. In the early days of chemical pesticides a century ago, non-biodegradable inorganic chemicals containing toxic elements such as arsenic, mercury and lead were often sprayed in high concentrations over food crops to control pests.
The impact on human health from the use of these pesticides remains disputed, with some studies suggesting that even for these compounds the doses of residue in food were too low to have any significant impact (Krieger 2005).
Nevertheless these compounds were largely replaced by much more effective and less toxic pesticides based on organic chemicals around the middle of the 20th century, and the use of pesticides based on toxic inorganic substances has subsequently been banned, or severely restricted, in most regions of the world (Peryea 1998).
Nevertheless many people still believe that modern day pesticides are still highly toxic and this view is perpetuated by certain NGOs (see Box 1 below).
Organic farming eschews the use of chemical pesticides of all types but grudgingly accepts that in some situations there is no practical alternative (Soil Association 2011):
“Occasionally, despite the best efforts of the farmer, problems may arise that can't be solved by using the practices that we recommend.... Under these circumstances there are some pesticides that can be used by the farmer as a last resort.”
When pesticides have to be used, the only ones allowed are from natural sources: extracts of natural products, live bacteria, or naturally occurring minerals.
This is ideological dogma, since in many cases these natural pesticides are more toxic and/or more environmentally damaging, not to mention far less effective, than modern day synthetic pesticide alternatives.
One example of this is the use of copper based fungicides, which are generally rather toxic relative to synthetic fungicides (tribasic copper sulfate is more toxic to rats than paraquat) and are also obviously not broken down in the environment and hence present a persistent threat.
Furthermore copper based fungicides are generally applied at a rate of several kilograms per hectare which is enough to turn sprayed foliage and fruit blue (Fig. 3).
By comparison, modern day synthetic fungicides are generally less toxic, less environmentally persistent and are more effective even when applied at concentrations ten times lower.
Another example is nicotine which may be used as an insecticide in organic farming in some parts of the world in the form of “tobacco tea” (IFOAM 2011). Nicotine, with a rat acute oral LD50 of 50 mg/kg (Science Lab 2011c; Oxford Univ. 2011d), is more toxic than all but two of the world’s best selling synthetic pesticides.
A commonly misunderstood concept is the Maximum Residue Level (MRL). It is often considered to be an absolute safety standard and hence food containing pesticide residues exceeding the MRL is considered dangerous to eat.
In fact the MRL is not a safety standard: it is a production standard intended to facilitate international trade and is simply an indicator of whether or not the pesticide has been used in accordance with the manufacturers’ recommendations.
The MRL is also generally set at the 95th percentile. In other words if the pesticide has been used in accordance with the instructions, 95% of treated crops will have residue levels at or below the MRL.
This also means that even if the pesticide has been used as instructed, it is to be expected that about 5% of treated crops will contain residue levels above the MRL. In fact the proportion of MRL exceedances is generally somewhat lower, as many farmers will not apply the maximum allowable amount of pesticide, nor leave the minimum allowable time between application and harvest (assumptions which are made in the definition of the MRL).
This is indeed what is observed: in a comprehensive survey of pesticide residues in food in Europe in 2008, 96.5% of over 70 000 foodstuffs tested contained residue levels at or below the MRL (European Food Safety Authority 2010) and in fact the survey actively targeted foodstuffs where it was felt that MRL exceedances would be most likely.
However this does not necessarily mean that the remaining 3.5% of foodstuffs containing residues exceed- ing the MRL present a significant risk to the consumer.
Further analyses showed that less than 0.2% of the foodstuffs tested had the potential to deliver doses of pesticide exceeding the “acute reference dose” (see Glossary) to individuals consuming very high quantities (> 97.5th percentile of general consumption) of the food in question.
Even then the acute reference dose is only 1% of the highest dose shown to be harmless to animals in controlled trials. The risk of acute poisoning by pesticide residues in food is vanishingly low.
But what about the risk of chronic health effects as a result of long term, low level exposure to pesticides? For every pesticide, long term animal studies must be conducted to define an “Acceptable Daily Intake” (ADI, see Glossary).
The ADI is typically set between 0.1% and 1% of the “No Observed Adverse Effect Level” (NOAEL see Glossary).
A set of highly conservative assumptions are then made about the exposure of consumers to the pesticide in food (for example, the pesticide will achieve 100% market share on every crop for which it is approved, all uses of the pesticide are at the maximum application rate and minimum time before harvest and no degradation of residues occurs during storage, processing or cooking).
In fact these assumptions are so conservative that in practice it is very difficult to consume doses of residue anywhere near the ADI for any given pesticide.
A comprehensive survey by the New Zealand government in 2003/4 showed that for over 90% of pesticides, typical dietary exposure is less than 0.1% of the ADI (NZFSA 2005).
Similar results have been reported from Australia (FSANZ 2003). This means that human dietary exposure levels are therefore generally at least 100 000 to 1 000 000 times lower than the NOAEL.
It should be noted that all concentrated formulations of pesticides should be treated with extreme care. Formulations are designed to optimise the uptake of the pesticide into biological organisms but they can also facilitate uptake into humans through skin contact.
The lack of appropriate protective equipment or carelessness when handling concentrated formulations can lead to accidental exposure corresponding to potentially toxic doses.
Data from the WHO indicate that acute poisoning owing to accidental exposure to pesticides is a major problem in the developing world.
Precise numbers are difficult to obtain but the indications are that over a million cases of accidental pesticide poisoning occur annually and the real figure could be many times higher than this (Jeyaratnam 1990; WHO 1990).
In contrast there are relatively few reports of accidental exposure in the developed world. Between 1945 and 1989 in England and Wales only 0.31% of total fatal poisonings were due to accidental exposure to pesticides (Casey and Vale 1994).
This indicates that it is generally possible to use these compounds safely so long as care is taken and adequate control measures are in place.
Whilst the focus of this paper is on the human toxicological consequences of consuming pesticide residues in food, it should also be noted that modern day pesticides are also rigorously tested to ensure that the impact of their use on the environment is acceptable.
This includes their toxicity to non-target organisms, for example earthworms and pest predators in the field, pollinator species such as honey- bees, aquatic organisms such as fish and prey species at the bottom of the food chain such as water fleas (Daphnia).
It is also critically important to assess how long pesticides survive in the environment, how likely they are to move through the soil and get in to groundwater, and to understand their metabolic fate and the impacts of potential metabolites (UK Health and Safety Executive website).
The total cost of the environmental and dietary safety testing that must be carried out by law on each pesticide is of the order of £100 million.
How naturally toxic is our food?
Every mouthful of food that we eat contains thousands of natural chemicals.
Many of these chemicals are more toxic than modern day pesticides and are present in food at higher concentrations.
This has been the case since humans first evolved and we have therefore evolved efficient mechanisms to deal with low concentrations of mildly toxic materials ingested in our diet.
A good example is the glycoalkaloid content of potato. Solanine is one of the main toxic glycoalkaloids produced by potatoes, and it is comparable in toxicity to the more toxic major pesticides (rat acute oral LD50 of 590 mg/kg (Swinyard and Chaube 1973)).
However solanine is found in potatoes in vastly higher concentrations than pesticide residues.
Depending on the variety, solanine le els can easily exceed 50 mg/kg of potato even after peeling (Friedman et al. 2003), and if the potatoes are allowed to “green” in light then the solanine levels can triple (Dao and Friedman 1994), reaching potentially toxicologically relevant doses in people eating even moderate quantities.
Another example is the psoralens found in celery. Psoralens are toxins that are activated by light. In the absence of light they are reasonably toxic (rat acute oral LD50 of 300-600 mg/kg), but in the presence of light they are far more poisonous: as little as 1 mg per kilo of body mass can be dangerous to humans (Capinera 2006).
In one reported case a woman ate approximately 450g of celery about an hour before visiting a suntan parlour. She suffered a severe, generalised phototoxic reaction resulting in serious blistering of the skin. Subsequent analysis suggested that she had consumed a dose of approximately 45 mg of psoralens in the celery (Ljunggren 1990).
Pesticide residues and food safety
Pesticide exposure is often “linked” in the media to serious medical conditions.
The evidence for these linkages only comes from studies involving high dose exposure: either animal studies or reports of human exposure to concentrated pesticide formulations.
It is scientifically unjustifiable to extrapolate from high dose studies to the ultra low dose exposures that are present in our diet (Ames et al. 1987).
There is no good evidence that exposure to pesticide residues in food increases the incidence of these diseases.
In fact if anything cancer rates have been declining during the period that synthetic pesticides have become widely used, perhaps owing to improving diets as food becomes more affordable (Trewavas 2004).
The use of pesticides in agriculture makes the resulting foods safer to eat, not more dangerous.
Firstly an innate resistance to pest attack in crops is an important component of pest control but a significant reduction, or elimination, of the use of pesticides will require the development of highly pest resistant crop varieties.
This begs an obvious question: why are some varieties of crop more resistant to pests than others? The answer is often that resistant crops produce higher concentrations of more noxious natural chemicals as their own internal pesticides.
In some cases, plant breeding programmes designed to develop more pest resistant varieties of crops have inadvertently led to increasing the concentration of such compounds to dangerous levels.
A good example of this is again that of psoralens in celery: a major grower developed and introduced a new, highly insect resistant strain of celery into the market which caused rashes on people who handled the crop and were then exposed to light.
The insect resistant celery was found to contain concentrations of psoralens nearly eight times normal levels (Ames and Gold 2000).
These natural pesticidal compounds produced by crop plants have obviously not been optimised to have low mammalian toxicity like modern synthetic pesticides.
In fact in most cases these compounds have not yet even been identified, let alone tested for toxicity. Moreover, it has been shown that the production of natural toxic chemicals in crops can be induced by pest attack, significantly increasing their concentration in food (Hlywka et al. 1994), emphasising the importance of crop protection for food safety.
The health consequences of eating a diet rich in crops with intrinsically high levels of pest resistance are unknown.
Secondly it is often forgotten that in the days before pesticides, food was often contaminated with high concentrations of extremely toxic natural chemicals that poisoned huge numbers of people.
Some of the most poisonous substances known to science are mycotoxins produced by fungi that grow on food crops. For example aflatoxins are the most potent carcinogens known to science.
The consumption of aflatoxin contaminated maize killed 80 people in Kenya in 2004 and hospitalised 180 others (BBC 2004). The condition known as “St. Antony’s fire” caused by consumption of ergot alkaloids in rye was very common in the Middle Ages, with 132 recorded epidemics in Europe between the 6th and 18th centuries.
Ochratoxins are produced by fungus that can grow on food in storage, and can cause aggressive cancers of the bladder and urinary tract (Bennett and Klich 2003).
Fusarium is a fungus that produces a cocktail of toxins which can contaminate wheat grain, one of which (fumonesin) has the potential to liquefy the brain tissue of horses eating contaminated feed (Dutton 2009)!
The elimination of these toxins from our diet relies upon effective control of not only the toxin-producing fungi, but also insects which can damage plant tissue facilitating secondary fungal attack.
The use of effective modern day fungicides and insecticides is the most certain way of achieving this.
Conclusions
Modern day pesticides are much less toxic than is commonly thought. They are comparable in toxicity to more familiar substances, such as over-the-counter medicines and common salt.
They are less toxic and are found in lower concentrations than many compounds found naturally in food.
Nevertheless modern pesticides are often erroneously thought to be deadly toxins, negatively impacting human health owing to the presence of residues in food and this view is deeply ingrained in the public consciousness.
Most people do not realise that the amount of pesticide residue to which they are exposed is many orders of magnitude too low to have any impact on their health whatsoever.
However, today in the developed world it seems that no levels of risk in our daily lives are deemed acceptable. Unachievable absolute guarantees of 100% safety are demanded and it is increasingly being proposed that the dominant paradigm by which new technology should be regulated is the precautionary principle (Pesticide Action Network 2011).
This would lead to technologies being banned even in the absence of a credible causal link between the technology and any purported detrimental side effects, and irrespective of the benefits that the technology may bring.
The essential benefits pesticides bring to global agriculture and food production are not widely appreciated. If pesticides are as dangerous and unnecessary as they are widely portrayed, then why are they used almost universally in commercial agriculture? The public seem unable to reconcile this contradiction.
Several NGOs and other groups with a “green” political agenda have been very effective in influencing public opinion against the use of pesticides.
This is in turn is driving changes in the legislation of pesticides within Europe and elsewhere that is making life difficult for farmers, whilst providing no benefits to consumers in terms of increased food safety.
Indeed this could lead to increased levels of natural toxins in food and an increased reliance on food imported from outside Europe where there is less control over production methods.
It is also pandering to the growing anti-science agenda in the developed world: a rejection of scientific rationalism in favour of a kind of holistic, alternative naturalism as the dominant philosophy.
Such modes of thinking are generally deeply suspicious of science and technology, at worst rejecting it altogether, dismissing any positive contributions and amplifying the potential risks.
In a world where it is critically important to inspire current and future generations of scientists to dedicate their professional lives to rising to the challenges facing humanity, such attitudes are profoundly unhelpful.
References
ADAS (2008) Evaluation on the impact on UK agriculture of the proposal for a regulation of the European Parliament and of the council concerning the placing of plant protection products on the market. European Crop Protection Association, Brussels. http://www.adas.co.uk/LinkClick.aspx?fileticket=BOSPfOEtvRs%3D &tabid=246 accessed 25 January 2011.
Ames, B.N., Magaw, R. and Gold, L.S. (1987) Ranking possible carcinogenic hazards. Science, 236, 271-280.
Ames, B.N. and Gold, L.S. (2000) Paracelsus to parascience: the environmental cancer distraction. Mutation Research, 447, 3-13.
Battisti, D.S. and Naylor, R.L. (2009) Historical warnings of future food insecurity with unprecedented seasonal heat. Science, 323, 240-244.
BBC (2004) Killer maize sparks Kenya alarm. http://news.bbc.co.uk/2/hi/africa/3802699.stm accessed 26 January 2011
Bennett, J.W. and Klich, M. (2003) Mycotoxins. Clinical Microbiology Reviews, 16 (3), 497-516.
Capinera, J.L. The Encyclopedia of Entomology, Volume 1, Dordrecht, Springer, 2006, p1557, ISBN 0 79 238 670 1. Available at: http://books.google.co.uk/ , accessed 26 January 2011).
Casey, P. and Vale, J.A. (1994) Deaths from pesticide poisoning in England and Wales: 1945-1989. Human and Experimental Toxicology, 13 (2), 95-101.
Cordell, D., Drangert, J.-O. and White, S. (2009) The story of phosphorus: global food security and food for thought. Global Environmental Change, 19 (2), 292-305.
Dao, L. and Friedman, M. (1994) Chlorophyll, chlorogenic acid, gly- coalkaloid, and protease inhibitor content of fresh and green potatoes. Journal of Agricultural and Food Chemistry, 42, 633-639.
Denholm, I., Devine, G.J. and Williamson, M.S. (2002) Insecticide resistance on the move. Science, 297, 2222-2223.
Dutton, M.F. (2009) The African Fusarium/maize disease. Mycotoxin Research, 25, 29-39.
European Food Safety Authority (2010) 2008 Annual Report on Pesticide Residues according to Article 32 of Regulation (EC) No 396/2005. European Food Safety Authority Journal, 8 (6), 1646.
FAO (2011) Food and Agriculture Organisation of the United Nations. http://faostat.fao.org accessed 26 January 2011.
Fisher Science (2011) Material Safety Data Sheet: Caffeine, Anhydrous, U.S.P./N.F. https://fscimage.fishersci.com/msds/ 95940.htm accessed 26 January 2011.
FSA (2008) Consumer Attitudes to Food Standards: Wave 8 UK Report Final. Food Standards Agency, London.
FSANZ (2003) The 20th Australian Total Diet Survey, Food Standards Australia New Zealand, Canberra, Wellington, ISBN 0 64 234 591 0. http://www.foodstandards.gov.au accessed 26 January 2011.
Foresight (2011) The future of food and farming. The Government Office for Science, London.
Friedman, M., Roitman, J.N. and Kozukue, N. (2003) Glycoalkaloid and calystegine contents of eight potato cultivars. Journal of Agricultural and Food Chemistry, 51, 2964-2973.
Godfrey, H.C.J., Beddington, J.R., Crute, I.R., Haddad, L., Lawrence, D., Muir, J.F., Pretty, J., Robinson, S., Thomas, S.M. and Toulmin, C. (2010) Food security: the challenge of feeding 9 billion people. Science, 327, 812-818.
Green, R.E., Cornell, S.J., Scharlemann, J.P.W. and Balmford, A. (2005) Farming and the fate of wild nature. Science, 307, 550-555.
Hall, R.L. (1992) Toxicological burdens and the shifting burden of toxicology. Food Technology, 46 (3), 109-112.
Hlywka, J.J., Stephenson, G.R., Sears, M.K. and Yada, R.Y. (1994) Effects of insect damage on glycoalkaloid content in potatoes (Solanum tuberosum). Journal of Agricultural and Food Chemistry, 42, 2545-2550.
Hodgson, J.A., Kunin, W.E., Thomas, C.D., Benton, T.G. and Gabriel, D. (2010) Comparing organic farming and land sparing: optimizing yield and butterfly populations at a landscape scale. Ecology letters, 13 (11), 1358-1367.
HSE (2011) UK Health and Safety Executive Chemicals regulation Directorate (CRD) Home Page. http://www.pesticides.gov.uk/ accessed 26th January 2011
IFOAM (2011) Criticisms and Frequent Misconceptions about Organic Agriculture: The Counter-Arguments. http://www.ifoam.org/growing_organic/1_arguments_for_oa/crit- icisms_misconceptions/misconceptions_no7.html accessed 14 February 2011.
Jeyaratnam, J. (1990) Acute pesticide poisoning: a major global health problem. World Health Statistics Quarterly, 43 (3), 139-144.
Krieger, R. (2005) Reviewing some origins of pesticide percep- tions. Outlooks on Pest Management, 16 (6), 244-248.
Ljunggren, B. (1990) Severe phototoxic burn following celery ingestion. Archives of Dermatology, 126, 1334-1336.
Merotto Jr., A., Jasieniuk, M., Osuna, M.D., Vidotto, F., Ferrero, A. and Fischer, A.J. (2009) Cross-resistance to herbicides of five ALS- inhibiting groups and sequencing of the ALS gene in Cyperus dif- formis L. Journal of Agricultural and Food Chemistry, 57, 1389-1398.
Nelson, G.C., Rosegrant, M.W., Koo, J., Robertson, R. et al. Climate change: impact on agriculture and costs of adaptation. Washington D.C., International Food Policy Research Institute, 2009, ISBN 9 780 896 295 35-3.
NZFSA (2005) 2003/04 New Zealand total diet survey: agricultur- al compound residues, selected contaminants and nutrients, Wellington, New Zealand Food Safety Authority, ISBN 0 47 829 801 3. www.nzfsa.govt.nz accessed 26 January 2011.
NHS (2011), How much salt is good for me? http://www.nhs.uk/chq/Pages/1138.aspx?CategoryID=51&SubCat egoryID=167 accessed 26 January 2011.
Oerke, E.-C., Dehne, H.-W., Schönbeck, F. and Weber, A. Crop pro- duction and crop protection: estimated losses in major food and cash crops, Amsterdam, Elsevier, 1994, ISBN 0 44 482 095 7.
Oerke, E.-C. (2006) Crop losses to pests. Journal of Agricultural Sciences, 144, 31-43.
OneWorld website (2011) http://us.oneworld.net/node/ 152674/ accessed 26 January 2011.
Oxford Univ (2011a) Safety data for ibuprofen. http://msds.chem.ox.ac.uk/IB/ibuprofen.html accessed 26 January 2011.
Oxford Univ (2011b) Safety data for caffeine. http://msds.chem.ox.ac.uk/CA/caffeine.html accessed 26 January 2011.
Oxford Univ (2011c) Safety data for sodium chloride. http://msds.chem.ox.ac.uk/SO/sodium_chloride.html accessed 26 January 2011.
Oxford Univ (2011d) Safety data for nicotine. http://msds.chem.ox.ac.uk/NI/nicotine.html accessed 14 February 2011.
Peryea, F.J. (1998) Historical use of lead arsenate insecticides, resulting soil contamination and implications for soil remediation. Proceedings of the 16th World Congress of Soil Science, Montpellier, France, 20th-26th August 1998.
Pesticide Action Network (2011) Promoting precaution. http://www.pan-uk.org/pestnews/Issue/pn53/pn53p10.htm accessed 2 February 2011.
Phillips McDougall (2010) Agriservice, Products Section – 2009 market. http://www.phillipsmcdougall.com accessed 19 January 2011.
Pivonia, S. and Yang, X.B. (2006) Relating epidemic progress from a general disease model to seasonal appearance time of rusts in the United States: implications for soybean rust. Phytopathology, 96 (4), 400-407.
Rickard, S. (2010) The value of crop protection: an assessment of the full benefits for the food chain and living standards, Crop Protection Association. http://www.cropprotection.org.uk/con- tent/home.asp accessed 19 January 2011.
Ridoutt, B.G. and Pfister, S (2010) Reducing humanity’s water footprint. Environmental Science and Technology, 44, 6019-6021. Royal Society. Reaping the benefits: Science and the sustainable intensification of global agriculture, London, The Royal Society, 2009, ISBN 9 78 085 403 784 1.
Sanchez, P.A. and Swaminathan, M.S. (2005) Cutting world hunger in half. Science, 307, 357-359.
Science Lab (2011a) Ibuprofen MSDS. http://www.sciencelab.com/msds.php?msdsId=9924344 accessed 26 January 2011.
Science Lab (2011b) Sodium chloride MSDS. http://www.sci- encelab.com/msds.php?msdsId=9927593 accessed 26 January 2011.
Science Lab (2011c) L-Nicotine MSDS. http://www.sciencelab.com/msds.php?msdsId=9926222 accessed 14 February 2011.
Soil Association (2011) Pest control: How organic farming avoids pesticides. http://92.52.112.178/web/sa/saweb.nsf/ ed0930aa86103d8380256aa70054918d/67fd448ec0643610802571 49004cb42d?OpenDocument accessed 26 January 2011.
Swinyard, C.A. and Chaube, S. (1973) Are potatoes teratogenic for experimental animals? Teratology, 8 (3), 349-358.
Thomas, R.J. (2008) 10th Anniversary Review: Addressing land degradation and climate change in dryland agroecosystems through sustainable land management. Journal of Environmental Monitoring, 10, 595-603.
Tilman, D., Cassman, K.G., Matson, P.A., Naylor, R. and Polasky, S. (2002) Agricultural sustainability and intensive production prac- tices. Nature, 418, 671-677.
Tomlin, C.D.S. (ed.) The Pesticide Manual, 15th Edition, Alton, British Crop Protection Council, 2009, ISBN 9 78 190 139 618 8.
Trewavas, A. (2004) A critical assessment of organic farming- and-food assertions with particular respect to the UK and the potential environmental benefits of no-till agriculture. Crop Protection, 23, 757-781.
Waring, W.S., Laing, W.J., Good, A.M. and Malkowska, A.M. (2009) Acute caffeine ingestion: clinical features in patients attending the Emergency Department and Scottish Poison Centre enquiries between 2000 and 2008. Scottish Medical Journal, 54 (4), 3-6.
WHO (1990), Public health impact of pesticides used in agricul- ture, Geneva, World Health Organisation, 1990, ISBN 9 24 156 139 4.
Wikipedia (2011a) Paracelsus. http://en.wikipedia.org/wiki/Paracelsus accessed 26 January 2011. I Wikipedia (2011b) Caffeine. http://en.wikipedia.org/wiki/Caffeine accessed 26 January 2011.
Zhang, W., Olson, E., Saintenac, C., Rouse, M., Abate, Z., Jin, Y., Akhunov, E., Pumphrey, M. and Dubcovsky, J. (2010) Genetic maps of stem rust resistance gene Sr35 in diploid and hexaploid wheat. Crop Science, 50, 2464-2474.
Acknowledgements
The author would like to thank Dr. Ray Elliott, Dr. Mike Bushell, Dr. Neil Lister, Judy Hughes and Jill Hughes for their help and suggestions in the preparation of this manuscript.
Download pdf
Figures
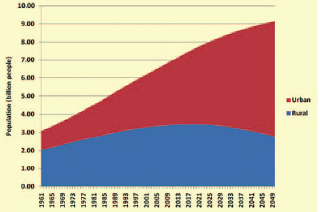
Fig. 1: Historical and projected human population (Source: FAO)
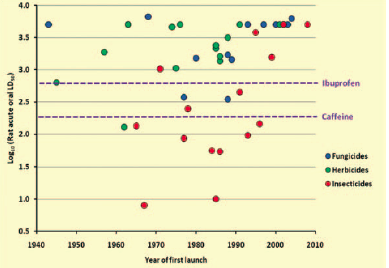
Fig. 2: Toxicity of the world’s best selling pesticides in 2009 plotted against their year of first introduction (Sources: The Pesticide Manual (Tomlin 2009) and Phillips McDougall 2010). The graph shows the toxicity of the 15 best selling her- bicides, the 15 best selling insecticides and the 14 best selling fungicides. The lower the number on the vertical axis (ordinate) the greater the toxicity, so toxicity is increasing down the graph. (N.B. all “copper-based fungicides” are omitted from the analysis because the sources considered them as a single entry, whereas in fact the different forms of copper used as fungicides exhibit a wide variation in toxicity, see Plate 1). Where toxicity is given as a range, or a difference is observed between male and female rats, the most toxic value is used, hence the graph portrays a worst case scenario.
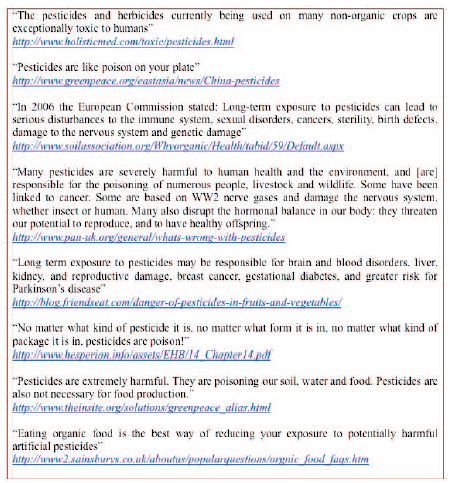
Box 1: Quotes from websites regarding the toxicity of pesticides (all accessed 27th January 2011
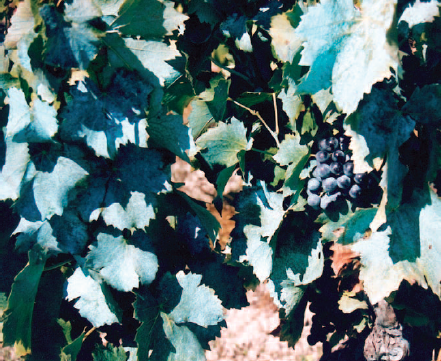
Plate 1: Grapes treated with copper based fungicide in the Côte du Rhône region of France, showing blue-green copper deposits on the fruit and leaves (photo kindly provided by Robert Cook)
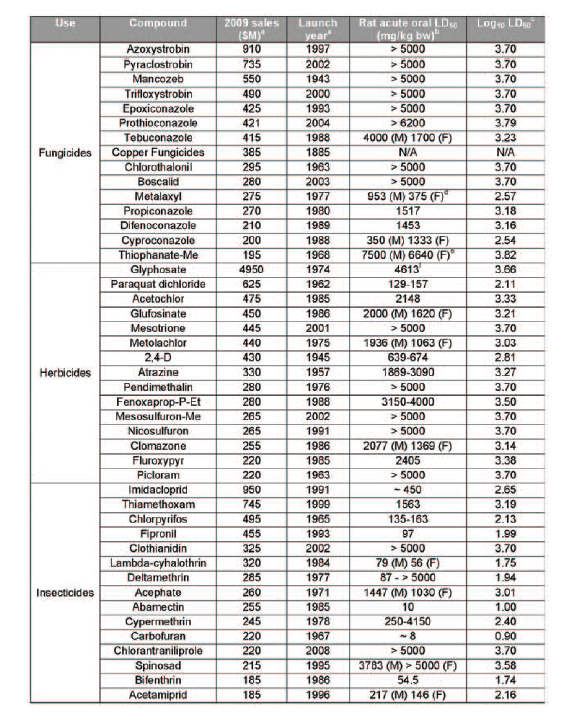
Table 1: Toxicity of the world’s best-selling pesticides in 2009. (Original data for those presented in Figure 2)
a Data taken from Phillips McDougall 2010.
b Data as reported in The Pesticide Manual (Tomlin 2009).
c Logarithm of the lowest value (either of the range or male/female) of the LD50 data.
d Toxicity data for metalaxyl-M.
e Toxicity data for thiophanate-methyl.
f Toxicity data for glyphosate-ammonium.